The Most Powerful Idea in the World Part 9
You’re reading novel The Most Powerful Idea in the World Part 9 online at LightNovelFree.com. Please use the follow button to get notification about the latest chapter next time when you visit LightNovelFree.com. Use F11 button to read novel in full-screen(PC only). Drop by anytime you want to read free – fast – latest novel. It’s great if you could leave a comment, share your opinion about the new chapters, new novel with others on the internet. We’ll do our best to bring you the finest, latest novel everyday. Enjoy!
The best known of them, Paul Romer of Stanford, made his reputation by demonstrating that useful knowledge-the largest component in Solow's residual, and therefore the most important component of any increase in national wealth-doesn't acc.u.mulate by itself, independent of the larger economy, but rather is almost entirely dependent on decisions made by individuals seeking some sort of economic advantage. Romer showed that such growth depended on real people acting, in their perceived self-interest, to create knowledge. His model was both stable and reflective of the real experience of economic growth since 1800. It also resolved, in a mathematically rigorous manner, the conundrum in Solow's fundamental growth equation,10 which was precisely how the initial stage of knowledge creation benefits the investor/inventor and therefore is counted in the "capital growth" segment, while the larger sum spills over into the economy at large and forms the residual.
More important, Romer demonstrated that the growth in useful knowledge occurred at anything but a constant rate; that it was, instead, highly sensitive to the economy in which it occurred. Romer recognized that the creation of the idea behind a new invention, or product, was just another fixed cost, like constructing a building, or buying a machine; it was just as expensive in skull sweat for James Watt to design the first Watt linkage as to manufacture a thousand. Because knowledge is the sort of property that can be sold to multiple consumers without lowering the value to any of them-Romer termed it nonrivalrous, as distinct from tangible, or rivalrous, property, which can be sold only once-the payoff for anyone producing it can be very large indeed.
At least, in a large enough population.
IN 1993, A DEVELOPMENT economist named Michael Kremer published a paper in the Quarterly Journal of Economics that examined population growth over time and tracked it against the expansion of what Romer called nonrivalrous, useful knowledge, and concluded that the creation of knowledge is directly proportional to the size of the population. Kremer's model made two a.s.sumptions:11 first, that inventive talent and motivation are randomly distributed throughout any population; and, second, that the larger the population, the larger the total output of inventions. The idea-that since each person has the same likelihood as any other of inventing the wheel, or the steam engine, or the iPod, more people means more inventors-may seem counterintuitive; it took only one genius, after all, to put stirrups on a saddle. But it works. A nation needs only twelve players to field a team to compete for the Olympic gold medal in basketball, and even tiny nations would find such a number easy enough to a.s.semble. But only a few nations are actually compet.i.tive, because the twelve players represent the top of a pyramid of compet.i.tion in which the larger the base, the greater the height.*
Thus, from the first century, when the world's population was around 128 million and its GDP about $105 billion,* to 1500, when population was 438 million and GDP $238 billion, the various growth equations demonstrate that all of the components of economic growth-land per worker, capital per worker, and therefore the residual-increased at a constant rate. More workers, more income, and, by inference, more invention. Moreover, the country-by-country numbers are relatively close in per capita terms; in 1500, each of the 48 million residents of Western Europe was producing, on average, about $772 annually, while China's 103 million were good for about $600 a head.
The year 1500 is significant because it's the one that marks what the economic historian Kenneth Pomeranz calls "the Great Divergence." Up until 1500, the difference between GDP per capita in the world's poorest countries and the richest was in general less than 50 percent; by 1820, it was more than 300 percent. After 1500, the lockstep increases in population and knowledge ended. From 1500 to 1820, China's population nearly quadrupled, and so did its GDP, with no increase in per capita GDP. During the same period, the population of France doubled, but its per capita GDP increased 56 percent; that of Great Britain quintupled, and its per capita GDP increased nearly two and a half times.
Kremer's theory was intended to explain productivity growth over a very long time frame; his article was ent.i.tled "Population Growth and Technological Change: One Million B.C. to 1990." However, for growth and change after 1500, and especially after 1700, it presents a pretty serious problem for the idea that inventiveness is directly proportional to population. Over the last five centuries, the theory only works by a.s.suming that one can usefully calculate an average for the entire world; but, especially since 1820, the worldwide growth rate has been severely distorted by the huge acceleration in productivity in Europe and North America, which is a little like calculating the average wealth of the patrons in a restaurant before and after Bill Gates enters it.
It's not as if Kremer was unaware12 of the problem; he acknowledged that for centuries, poor but populous countries like China and India had experienced decidedly low research productivity, which at least suggested that inventiveness is a function of income rather than population, but not enough to change his basic thesis. That thesis, however, is considerably more persuasive when the time span is more than a million years than when one is examining a single century or a single country. But if a large population alone doesn't improve the chances of the steam engine's being invented in any particular nation, what does? Why did none of China's 138 million people invent a working steam engine, while Thomas Newcomen, one of fewer than nine million Britons, did?
China's size, its extraordinary technological head start, and its relative isolation have made it, for centuries, an irresistible laboratory for theorizing about industrialization and invention. Most of the resulting theories end up emphasizing either China's geography, science and technology, demography, or political culture.
Geography first. Geographical determinism is the notion that mineral resources, topography, and climate are the key drivers of human history. It remains eternally popular as an explanation for everything from political conflict to pandemics; but China's missing Industrial Revolution looks like a poor example of it. Pomeranz attributes a good deal of his "Great Divergence" to the relative ease with which British coal was extracted and therefore iron produced. But China had, and has, huge coal deposits,13 just as close to the surface, and China's forges were using coal to produce as much iron in the year 1080 as all of Europe did seven centuries later. They even did so for the same reason: the Yangtze delta had been deforested by the same demands as were the English Midlands, namely, construction, heating, and smelting. And even though the barbarian invasions14 of the twelfth and thirteenth centuries pushed China's center of gravity south to less coal-rich areas (China's nine southern provinces have less than 2 percent of contemporary China's coal reserves), iron production rebounded almost immediately and by 1700 was certainly larger in China than Europe. Neither Europe nor Britain truly had, to an objective eye, any advantages in mineral wealth, climate, or navigable waterways.
The state of Chinese science relative to the West seems a more promising explanation than its geography. It seems plausible, for example, to argue that if Chinese scientists failed to uncover the foundation principles unearthed by Torricelli and Boyle, Chinese inventors would have found it far more difficult to replicate the work of Newcomen and Watt. This is a small part of what the eccentric Cambridge don Joseph Needham spent his life investigating and chronicling in the two dozen volumes of his masterwork, Science and Civilization in China. Needham's conclusion,15 unfortunately, doesn't do much to confirm this particular diagnosis: he argues that the basic understanding of both vacuum and adiabatic pressure-the phenomenon that causes a gas to cool when it expands and heat when it is compressed-was present in China from the thirteenth century onward. Even if this overstates the case, a reasonable consensus exists for the belief that by the sixteenth century China had enough awareness of atmospheric pressure to produce not only a Newcomen-style reciprocating steam engine, but one that produced rotary motion.
Chinese engineers had already shown remarkable cleverness in transforming rotary into reciprocating motion. By 400 CE they had developed a system of water "levers,"16 which used a waterwheel to fill a chute with water, tipping it first in one direction, then in another. Even more significant, and well known, was China's twist on the blast furnace, which, as the careful reader will recall, was being used to make cast iron in China by 200 CE, at least a thousand years before one appeared in Europe. The Chinese version of this device, called a box bellows, used a piston to pump air in and out of a cylindrical box. Early box bellows were hand-operated, but, in legend at least, sometime around 30 CE a Chinese inventor named Tu s.h.i.+h hooked the piston up to a waterwheel-driven crank, thus facilitating the transfer between reciprocating and rotary motion seventeen centuries before Watt and Pickard. But it was an English historian named Ian Inkster, writing in 1842, who realized the potential for a box bellows piston that produced force on both strokes, writing, "let it [the box bellows] be furnished17 with a crank and flywheel to regulate the movements of its piston, and with apparatus to open and close its valves, then admit steam through its nozzle, and it becomes the double-acting engine." Pomeranz agreed: "The Chinese had already recognized18 the existence of atmospheric pressure, and had long since mastered (as part of their "box bellows") a double-acting piston/cylinder system much like Watt's, as well as a system for transforming rotary motion to linear motion that was as good as any known anywhere before the twentieth century. All that remained was to use the piston to turn the wheel rather than vice versa."
That was all that remained. It still remained when the first steam engines in China were being imported from Britain. The box bellows was undeniably an inventive leap, but not in any useful direction. The bellows, it turns out, is not a mirror image of the piston, but its opposite. To the degree that it works as a bellows, it cannot work to drive a piston. The Chinese could have a bellows,19 or a vacuum, but not both, at least not without a strong theory of the behavior of gas and pressure like the one articulated by Boyle in the seventeenth century.
And they didn't have one. Chinese science had taken a very different path than its a.n.a.logue in the West; most especially it lacked the strong historical foundation-no Descartes, no Galileo, no Bacon-needed to develop the experimental science of the Enlightenment. Even worse: When a scientist like the polymath Fang Yizhy attempted to import Western methods into China in 1644, producing a mammoth collection of works on mathematics, engineering, and natural philosophy ent.i.tled The Small Encyclopedia of the Principles of Things, it lacked any readers.h.i.+p outside the aristocracy; China's master artisans were so severely handicapped by illiteracy20 that eighteenth-century Chinese literacy rates never got much higher than 40 percent, at a time when 60 percent of British men were able to read.
If the Chinese handicap was neither geographic nor scientific, was it demographic? Even with Chinese literacy rates barely two-thirds that of Britain's, by 1700 there were still more literate Chinese than the entire populations, literate and otherwise, of France, Germany, Italy, and Britain combined. How is it possible that not one of them was capable of inventing a spinning jenny? The only difference between Hargreaves's invention and the machine used by Chinese cotton spinners, for example, was the draw bar, a device whose "fingers" could pull a large amount of softly wound cotton. The draw bar was not a complicated device,21 and yet even though at least five times as many Chinese as Britons were spinning fibers into yarn, there is no evidence that any of them invented one.
Instead, it appears that China's huge population, and its powerful central government, both of which would seem to be advantages for industrialization, were liabilities when combined. The population's demands for food were a tiger by the tail for the imperial court for centuries; so many mouths to feed meant that the investment capital available demanded single-minded focus on agricultural innovation. Just as contemporary scientists and inventors "follow the money," writing proposals in the subjects that granting agencies currently favor, so Chinese innovation pursued those avenues for which support was available. The difference is that contemporary scientists can select from many patrons; in China there was only one.
As with Tudor England, government monopoly of patronage meant control. Virtually all copies of the seventeenth-century Chinese encyclopedia, the T'ien Kung K'ai-wu or Exploitation of the Works of Nature, which included ill.u.s.trations of everything from hydraulics to metallurgy, were destroyed because, according to Joseph Needham, much of the material touched on industries that had been granted monopoly status by the Qing emperors: "The absence of political compet.i.tion22 did not mean that technological progress could not take place, but it did mean that one decision-maker [i.e. the Emperor] could deal it a mortal blow." It is therefore no surprise that a high percentage of both the inventions and inventors we a.s.sociate with China from the time of the Han Dynasty to the Qings were government sponsored and employed.
Another liability of a strong central government is that it is, well, strong. Europe's fragmented system of sovereign states23 made it possible for innovative minds such as Paracelsus, Leibniz, Rousseau, and Voltaire to "shop" for more congenial places whenever they skated too close to heretical or otherwise challenging notions; in China, one had to travel a thousand miles to a place where the empire's writ ran not. And since China, perversely, was able to keep from plummeting down a Malthusian hole by using its enormous geographic extent to expand land under cultivation in the southern and western territories, technological stagnation, until contact with the West, seemed to have few if any costs.
In the end, however, neither territory, nor politics, nor even science is as powerful as culture in explaining China's inability to produce its own steam engines, puddling furnaces, or spinning jennys. Bertrand Russell translated the Chinese term24 wu wei (usually "doing without effort") as "production without possession"-a simplification, no doubt, but one with some powerful resonance to the notion that China's great burden was a lack of the itch to own one's own work.
ON THE OTHER HAND, that itch was powerfully felt in the seven United Provinces of the Netherlands from their de facto founding in 1581, when the onetime Spanish colonies abjured the rule of Philip II. By the beginning of the seventeenth century, the Netherlands was home to Europe's most cosmopolitan culture; the world's first stock market; a large and powerful merchant cla.s.s, including Europe's largest bankers; access to millions of consumers through its enormous merchant fleet and colonies; the rule of law; and tolerance for every religious confession in the world, including the best established and a.s.similated Jewish community in the world. It had developed, out of deference to the North Sea, a huge network of windmills, ca.n.a.ls, and waterwheels. It even had, from 1683 to 1688, the prophet of the concept of intellectual property, John Locke, who wrote most of the Treatise on Government while living in Utrecht, Linden, and Amsterdam.
And it had a deep respect for inventions and inventors. Corneliszoon's crank-operated sawmill was far from an unusual case. From 1600 to 1650, the Dutch government25 issued between five and ten technical patents annually. However, the pace thereafter dropped off precipitously-and the reasons are telling. Petra Moser, now a professor26 at MIT's Sloan School of Management, spent four years examining more than 15,000 different inventions exhibited at nineteenth-century world's fairs, and their equivalents, and discovered a fact that seems at first glance to discredit the idea that patent protection was essential for innovation: Nations without patent laws were in many cases just as inventive as those with them. Or even more inventive; some of the nations best represented at those industrial fairs actively discouraged the patenting of inventions.
The reason seems to be that whether or not they enforced a patent law, smaller nations or domains, such as the Netherlands and Switzerland, were vulnerable to the theft of their innovations by compet.i.tors in larger nations. The bargain of patent protection runs two ways: The state, in return for making an idea public, offers legal recourse to its creator should someone within the state steal the idea. Since making one's invention public in a nation with patent protection offered protection against theft only up to its own borders, only a large nation offered a large enough market to make the deal a good one, and (in Moser's words) the small nations "would have been silly27 to patent [their] innovations."
This logic inhibited investment in entire categories of innovation. Those nations that relied on secrecy rather than patent tended to specialize in the sort of inventions that cannot be easily reverse-engineered, such as chemicals or dyes. One consequence is that almost all mechanical inventions-particularly, all steam engine innovations-were produced in countries that enforced some sort of patent law, since one can scarcely sell a compound engine and simultaneously keep its workings secret. Another is that any benefit from the cross-fertilization of ideas resulting from the public requirement of patent law, including publication of specifications, was lost.
The result is that while the Netherlands led the world in per capita (really, per man-hour) GDP every year from 1700 to 1820, its average compound growth rate over that period was actually negative, falling more than 13 percent. From there on, Britain took over the lead,28 with a growth rate per man-hour of 0.5 percent-a rate that exploded to growth of 1.4 percent annually from 1820 to 1890. The remarkable growth of the Netherlands during the 1600s essentially stopped a century later, and the only persuasive reason is size, or rather scale. A small country can shelter the world's largest banks, s.h.i.+pbuilders, and even textile manufacturers, but since it can protect inventors only from their own countrymen, growth that depends on the creation of new knowledge is fundamentally unsustainable, like a nuclear chain reaction with insufficient critical ma.s.s. Just as fission requires that a sufficient number of uranium nuclei be, in some sense, accessible to the others, a chain reaction of innovation sustains itself only if innovations are accessible to one another. A few thousand Europeans, no matter how inventive their work in chemicals, or metallurgy, could not create an Industrial Revolution unless they could inspire (or borrow, or even steal) from one another; a few thousand Britons, precisely because they concentrated their efforts on "public" inventions, most especially the steam engine, emphatically could. The consequence is that smaller nations, by avoiding large-scale mechanical invention out of fear that their own territory was too small to make them profitable, deferred industrial leaders.h.i.+p to those large enough to take the risk.
So if the Netherlands was too small, and China too big,* what nation was, in the immortal words of Goldilocks, "just right"? The most intriguing candidate29 would seem to be France, which had just about every advantage that Britain had, and a lot more Frenchmen to exploit them. France's economy grew at almost precisely the same rate as Britain's between 1700 and 1780, and from a far larger base; only about a tenth of one percent per year less growth in industrial (and, for that matter, agricultural) output in France during those eight decades. In 1789, the year of the Revolution,30 France's foreign trade was 25 percent greater than Britain's, and its population was nearly three times bigger.
By the same year, however,31 Britain had a significant lead in any number of significant indices: a third more GDP per capita, far higher rates of urbanization (nineteen of every hundred Britons lived in cities, and only eight Frenchmen), nearly eight times as much money in the hands of banks, and a tax rate less than a third of France's. Thus, in part because of lower interest rates32 from the middle of the eighteenth century forward, the availability of capital (as opposed to its absolute amount) was significantly greater in Britain.
But it was not only, or even mostly, an advantage in business sophistication that gave Britain a head start on industrialization. Nor was it scientific sophistication, a yardstick on which France was way ahead: Watt was simultaneously a brilliant engineer33 and a gifted scientist, but he still needed to study French engineering texts because there were so few English ones available. His experience remained true through most of the nineteenth century, when French, and later German, scholars were giving a scientific foundation to the laws of thermodynamics and kinematics.
And they were doing so in an environment in which standardization was very highly valued, and strictly enforced. Possibly because it achieved status as a coherent nation-state centuries before England, to say nothing of Britain, France has a far longer history of activism in setting national standards; the Academie Francaise, as a case in point, has been protecting the purity of the French language since 1634. The project of synthesizing the knowledge held tightly in the hands of French artisans, mechanics, and craftsmen began only forty years later, when the Academie des Sciences-France's equivalent of the Royal Society-began a national "Description des arts et metiers" intended to establish standard versions of hundreds of apparatuses. Among other things, the project provided Diderot34 and his Encyclopedie with more than 150 drawings and engravings of water pumps, looms, and forges. In the following century, the French government explicitly took on the responsibility of educating and training engineers with the founding of several schools focused on applied science, including the ecole des Ponts et chaussees in 1774; the ecole polytechnique was founded by a graduate of Ponts twenty years later, in part to impose technical standards on industry with the same rigor that the Academie governed the French language. The Encyclopedie itself promised "to offer craftsmen the chance to learn35 from philosophers, and thereby hopefully to advance further toward perfection."
In Great Britain, on the other hand, inventions were much more of a haphazard process, performed by onetime wheelwrights and carpenters competing, rather than collaborating, with one another. Their success did not go unnoticed in France, nor unremarked. In 1824, an ecole polytechnique graduate named Sadi Carnot wrote Reflexions sur la puissance motrice du feu et sur les machines propres a developper cette puissance-the first theoretical explanation of the thermodynamics of steam power-essentially out of dismay that the great achievements of British engineering had been produced by men, like Watt, with no formal schooling. The sn.o.bbery served French science well; less well French innovation. If one secret to sustaining an inventive culture was making inventors into national heroes, it was a secret that didn't translate well into French. Between 1740 and 1780,36 the French inclination to reward inventors not by enforcing a natural right but by the grant of pensions and prizes resulted in the award of nearly 7 million livres-approximately $600 million today*-to inventors of largely forgotten devices, but Claude-Francois Jouffroy d'Abbans (inventor of one of the first working steamboats), Barthelemy Thimonnier (creator of the first sewing machine), and Aime Argand (a partner of Boulton and friend of Watt whose oil lamp became the world's standard) all died penniless. Other than Joseph-Marie Jacquard, the creator of the eponymous loom, and perhaps the Montgolfiers, the French did not lionize their inventors.37 This didn't mean they didn't understand the strategic importance of technology. Carnot himself wrote, "to deprive England of her steam engines,38 you would deprive her of both coal and iron; you would cut off the sources of all her wealth, totally destroy her means of prosperity, and reduce this nation of huge power to insignificance. The destruction of her navy, which she regards as the main source of her strength, would probably be less disastrous." Compet.i.tion with Britain alone might have allowed French industrialization to survive the haughtiness that made the nation elevate pure science over its commercial applications, if not for an unfortunate bit of timing. The same year that Joseph Bramah was hiring Henry Maudslay to help build his locks at 124 Piccadilly, several thousand citizens of Paris marched down the Rue Saint-Antoine to the nearly empty prison known as the Bastille. The same year that the British government was certifying a grant of incorporation for Boulton & Watt to design and sell steam engines, the French government was beheading Antoine Lavoisier, the chemist whose research on heat was central to the theory behind those engines.
It was not immediately apparent that the French Revolution would be hostile to invention or inventors. The first law protecting intellectual property in France was pa.s.sed in 1791, in ringing language that declared, every novel idea39 whose realization or development can become useful to society belongs primarily to him who conceived it, and that it would be a violation of the rights of man in their very essence if an industrial invention were not regarded as the property of its creator.
Unfortunately for the cause of innovation, the law was abrogated only two years later, as a side effect of the extreme violence of the Terror. And while it was reinstated in 1794, n.o.body seems to have told the French patent office. They were already playing catch-up in 1792, when Britain granted 85 patents, and France, with a population twice as large, issued 29. In 1793, that number fell to 4. From 1793 to 1800, in fact,40 Britain issued 533 patents to France's 65. In addition to all their other world-historical effects, the French revolutionists, and the Corsican emperor whose wars were the Revolution's last chapter, const.i.tute the most important reasons that Britain and America established a thirty-year lead on all other European nations in the development of steam power. As Jeff Horn, whose study of French industrialization is very close to the last word on the subject, put it, "When the revolutionary and Napoleonic wars ended41 in 1815, the British were approximately a generation ahead in industrial technology and in the elaboration of the mechanized factory."
Looked at through the history of ideas, the French att.i.tude toward invention, and even its revolutionary spirit, share a common origin. To the same degree that Britain's beliefs about property are traceable to John Locke's Second Treatise on Government, France's can be found in the Discourses of the onetime engraver, writer, musician, and philosopher Jean-Jacques Rousseau.* In his First Discourse, for example, Rousseau shared his discomfort with technical progress, which he a.s.sociated with decadence and moral decline; his Second Discourse argued that the invention of any technology, by demonstrating that some are more gifted than others, promotes inequality and eventually tyranny: "Astronomy was born from superst.i.tion ... physics from vain curiosity" (First Discourse, volume I). In his "treatise" on education, emile, Rousseau attacked what has come to be known as amour propre: the invidious striving after excellence in the eyes of one's fellows. Rousseau's fetish for compa.s.sion and equality have made him a powerful influence on generations of Marxists, but his earliest, and most consequential, impact was on the revolutionaries of eighteenth-century France. When in 1793 the Jacobins closed the Academie des Sciences on the logic that "the Republic does not need savants,"42 they were channeling Rousseau.
And they were hampering their Republic in the race to technological mastery. The finish line for the first stage of that race had been the use of condensed steam to convert atmospheric pressure into the reciprocating motion of Newcomen's pumps. For the second stage, it was converting the expansive power of steam into rotary motion able to drive dozens, and then hundreds, of spinning and weaving machines.
The third stage was converting steam power into motion. Locomotion.
* This is a particularly strong argument against a belief in progress.
* David Warsh points out, in his own Knowledge and the Wealth of Nations (which has inspired much of this chapter), that Smith's book was the first of only four dominant textbooks the field has ever known; the only one until Ricardo's Principles of Political Economy and Taxation, which was followed by Alfred Marshall's Principles of Economics and eventually by Paul Samuelson's Economics. The pattern of successively shorter t.i.tles seems finally to be at an end.
* In fact, the same process works in reverse, though at the expense of the pyramid metaphor. Not only does a larger population result in more inventions, the wealth created by those inventions permits even more population growth, and yet another Malthusian trap.
In units called Geary-Khamis dollars, each of which is equivalent in purchasing power to a U.S. dollar in 1990, and which is the benchmark "currency" of choice for really long time ranges.
* Perversely enough, nineteenth-century Russia was both too big and too small. In 1766, the brilliant Ivan Polzunov, inventor of the world's first two-cylinder engine, died of tuberculosis, and when his engine, which operated the bellows in Russia's largest forge, needed repair three months later, no one could be found who understood how to rea.s.semble it.
* It is even harder to calculate the current value of eighteenth-century French currency than British. From 1726 on, an ounce of gold was set equal to 92.5 livres; during the same period, an ounce of gold cost 4.10. Seven million livres, therefore, equaled about 310,000. Using the index of average earnings, this is more than 400 million today.
* The Locke versus Rousseau debate remains one of academe's almost preternaturally popular, used to explain everything from the differences between modern and medieval perspectives to the reason for Britain's reluctance to adopt the euro.
CHAPTER TWELVE.
STRONG STEAM.
concerning a Cornish Giant, and a trip up Camborne Hill; the triangular relations.h.i.+p between power, weight, and pressure; George Was.h.i.+ngton's flour mill and the dredging of the Schuylkill River; the long trip from Cornwall to Peru; and the most important railroad race in history THE CAUSE OF THE ACCIDENT at Poldory Mine in January 1784 is not precisely known, nor is its date; some accounts have it occurring on the sixteenth, others on the nineteenth. Its effect on the mining families of Cornwall's Gwennap Valley, about two miles from the town of Redruth, was depressingly routine: tragic for the six families that lost their breadwinners, a reminder to everyone else of the dangers of mining-cave-ins, floods, fires, and suffocation.
The risk of each hazard increased in direct proportion to the mine's depth, and by 1784, Cornwall's copper was being carved from seams several hundred feet below the surface. This made Poldory, and its neighbor, the Ale-and-Cakes,* utterly dependent on the Boulton & Watt engine pumping the water out of the two mines and into the Great County Adit that drained a good portion of central Cornwall. If the engine didn't work, neither did the miners.
The reliance on steam power, however, had introduced a new danger: catastrophic failure of the engine itself. Not all steam engine failures are catastrophic-complete and sudden. Valves can stick, or beams crack, harming only the engine itself. A failure of the engine's cylinder housing or boiler is different. The sudden release of steam under pressure is literally explosive, sending shards of metal flying outward at several hundred feet per second, driven by jets of scalding steam. It was precisely this sort of accident that killed three miners, and maimed several more, at Poldory, and when the news reached the engine's designer and builder, his reaction was predictable. On January 24, James Watt wrote to Thomas Wilson, a mine owner living in the town of Truro in Cornwall, I am exceedingly shocked1 at the account of the accident at Poldory and should have been Glad to have had some particulars. They must certainly have had a very strong steam otherwise, the people would have had time to escape. Please also to advise who the people were and how so many came to be about the boiler; Copper tubes must be entirely given up without men can be found more carefull [sic] in the management of them. If any of the families of the deceased or the surviving persons who were scalded are in distressed circ.u.mstances, I am sure that Mr. B[oulton] will Join me in being pleased that you should give a small matter for their immediate relief as if of your own accord without mentioning our names ..."
The phrase "strong steam" is telling. By 1784, Watt was decades removed from his first experience with Newcomen engines, but those decades had done nothing to ease his fear of the caged power of steam under pressure. Some of the concern dates to his earliest experiments: two years of sealing materials that failed to seal, tubing that leaked, and cylinders with seams that burst. The larger part, however, was an a.n.a.lytical blind spot, one that he shared with the most sophisticated scientists of the eighteenth century.
That blind spot, about the nature of the relations.h.i.+p between heat and motion, was no longer the belief that phlogiston was released whenever anything was burned. The failure of phlogiston theory to account for any number of observed phenomena had opened the door for another, more useful though still flawed, to take its place. This was the idea that heat remained a substance, but a weightless one, called caloric. The Scottish philosopher William Cleghorn, another protege of Joseph Black, theorized that this "subtle fluid" (in the words of Lavoisier, who also coined the word "caloric") was a gas whose properties included varying levels of attraction to different types of matter, thus explaining why the heat capacity of coal was different from that of gla.s.s. The theory further held that caloric could be neither created nor destroyed, but only changed from Black's latent heat-the potential locked up in a combustible substance-to sensible heat, and then back again, with the total amount of caloric in the universe staying constant.
Caloric theory remained the conventional wisdom for sixty years or more for a simple reason: It explained, better than any alternative, dozens of physical phenomena. Hot fluids cool, in caloric theory, because caloric repels itself, thus diffusing from an area of high concentration to a lower one. It explains heat radiation and Boyle's Law, and even formed the basis, forty years after Poldory, for Sadi Carnot's Reflexions, and the first working theory of steam engines: that their capacity depended only on the difference between high temperature and low temperature, which, in Watt's steam engine, was the difference between the temperature of the boiler and that of the condenser.
This didn't mean that no one was thinking outside the caloric box. There was, for example, the thoroughly remarkable Benjamin Thompson of Ma.s.sachusetts, a loyalist American who, after backing the losing side in the Revolutionary War, moved, first to England (where in 1779 he was made a Fellow of the Royal Society), and four years later, apparently on a whim, to Bavaria. There he found himself, on behalf of the Prince-Elector2 Karl-Theodor, running an espionage network that stole design sketches from the Soho Manufactory and spirited them out of England. For this and other services (including the invention of Rumford Soup, a concoction of peas, barley, potato, and old beer intended to meet the nutritional needs of Europe's poor) he was made a Count of the Holy Roman Empire in 1791.
Seven years later, Count Rumford (as he had styled himself, after the New Hamps.h.i.+re town where he had been a schoolmaster) performed an experiment investigating the nature of heat, inspired by his observation of Bavarian metalworkers boring out a bra.s.s cannon barrel, which generated enough heat from friction that the barrel was too hot to touch. Lavoisier's theory predicted that the caloric a.s.sociated with drilling should have melted the bra.s.s shavings into which it presumably had been transferred. Since it did not, Rumford tested it, rather cleverly, by boring a cannon barrel underwater. The borer's friction produced enough heat to keep the water boiling-and the water continued boiling as long as the borer was spinning, thus disproving the idea that caloric was a property contained within matter, since it was never exhausted.
If caloric was not a fluid, it had to be something else. Rumford's monograph, An Experimental Enquiry Concerning the Source of the Heat Which Is Excited by Friction, argued that the "something else" was actually motion: that heat and motion are essentially the same thing. This was critical, and surprisingly slow in coming. It is, after all, not hard to find places outside the laboratory where mechanical work creates heat: rubbing two Boy Scoutapproved sticks together, for example. John Locke himself observed the heat produced by the mechanical energy of a wheel rubbing against its axle.
But even though Count Rumford shot a very large hole in the idea of caloric, theories are overturned only by better theories, and he didn't really have one to offer. So long as caloric theory was still an effective way of approximating physical reality, it wasn't going away. This was a real obstacle to engineers, since a key element of caloric theory confused the nature of work in steam engines. Caloric theory held that heat was latent3 within combustible materials and was simply converted from latent to sensible, which "proved" that there could be no advantage to a high-pressure engine, which would simply increase sensible heat at the expense of latent heat.
This was confounded by another blind spot: Until the middle of the nineteenth century, scientists and engineers alike widely believed that the source of a steam engine's work was the pressure of expanding steam on a moving piston. More pressure, therefore, equaled more work. So far, so good. But the a.n.a.logy they used as a model was waterpower, and that was not so good. A waterwheel produces the same amount of work when relatively little water flows over a long distance as it does when a lot of water flows over a short distance; a waterwheel will make as many revolutions when it catches a trickle that has fallen a thousand feet as when it is driven by a river falling a few inches. By the same token, they reasoned, a steam engine would be just as efficient using low pressure to drive a long beam as high pressure to drive a shorter one-and steam, as the families of the Poldory miners would certainly testify, is a lot safer at low pressure.
Reasoning by a.n.a.logy is always suspect. What was missing in this example was an understanding that it was not the pressure that mattered, but the heat-heat energy and mechanical energy were just different forms of the same thing. The heat needed to raise one pound of water a single degree is equivalent to lifting 772 pounds one foot, and vice versa: lifting 772 pounds one foot generates an equivalent amount of heat.* The slow realization of this relations.h.i.+p is explained largely by the low efficiency of the early engines. If you're converting only 2 percent of the heat energy into work, you'd be hard pressed to notice its absence. And even when they noticed it, the blind spot endured. No matter how many times engineers observed4 more work being done with more heat, they were unable to make any sense of the results.
This blinkered view didn't change the need for steam engines to deliver more power, of course; water needed to be pumped from ever deeper mine shafts, factories needed more power to drive larger wheels. The response of Boulton & Watt was Archimedean: longer levers driven by ever larger condensing engines, using cylinders up to five feet in diameter, with strokes of nine or even ten feet. That these behemoths offered a pretty unattractive power-to-weight ratio didn't seem at the time to be much of a problem; low pressure-about 8 to 10 psi-was reliable, safe, and affordable. A multi-ton condensing steam engine could pull a bucket from a mine shaft five hundred feet deep, or run a dozen shafts at a cotton spinning factory. But it couldn't pull itself any distance at all.
It wasn't that no one thought about the possibility of steam locomotion. In 1784, only four months after Poldory, Watt himself described, in the same patent that included the parallel linkage, a piston-driven steam carriage that used "the elastic force of steam5 to give motion." In 1785, Boulton & Watt's brilliant engineer William Murdock actually built a "steam carriage" and for the first time filed a patent in his own name. He even built a scale model of his carriage-a cylinder with a diameter of three-quarters of an inch and a stroke of an inch and a half-and decided to exhibit it in London, though there is no reason to believe that it would have been a success. Asking a steam engine to move itself-to say nothing of cargo or pa.s.sengers-meant making it powerful and lightweight; and the only way to do that was increasing the pressure in the cylinder. With his fortune tied to the future of low-pressure steam power, Matthew Boulton himself intercepted Murdock6 on the way to London and persuaded him to return to building stationary Boulton & Watt engines in Cornwall.
Which was where, in any case, the next revolution in steam engines was going to occur. There, and in America.
BY THE MIDDLE OF August 1787, the fifty-five delegates to the world's first, and most consequential, Const.i.tutional Convention had been meeting in Philadelphia for three months of an extremely hot summer. They had debated judicial appointments, executive departments, and every conceivable duty of a national legislature. They had established the line of command for the new nation's armed forces and proposed a national postal service. Given all that, it's no great shock that the first acknowledgment that the newly proposed federal government had any place in protecting the activities of inventors didn't come until August 18, when James Madison of Virginia proposed that the national legislature be empowered to "encourage knowledge and discoveries." The same day, Charles Pinckney of South Carolina submitted a proposal that the government be able "to grant patents for useful inventions."7 Four days later, on the twenty-second, the convention adjourned for the afternoon and headed to the banks of the Delaware River to see a demonstration of the power of such useful invention: a forty-five-foot-long boat that resembled an Iroquois war canoe, with six oars on either side. The motive force for those oars, however, was not muscle. It was steam.
The steamboat's inventor, a onetime clockmaker and silversmith from Connecticut named John Fitch, had turned a traditional twelve-inch condensing cylinder on its side and used it to drive a piston with a three-foot stroke tied to an eighteen-inch axle. In his own words, "Each revolution of the axle tree8 moves twelve oars five and a half feet. As six oars come out of the water six more enter the water; which makes a stroke of about eleven feet each revolution. The oars work perpendicularly and make a stroke similar to the paddle of a canoe."
Fitch's steamboat was not, as many histories have it, the world's first. In 1772, two exartillery officers in the French army, the Comte d'Auxiron and Charles Monnin de Follenai, received a fifteen-year exclusive license to run a steamboat along the Seine. Unfortunately, their first attempt, a marriage of a Newcomen engine to a Seine bateau, was less than successful: the engine was so heavy it sank the boat. Slightly more successfully, in 1783, the Marquis de Jouffroy d'Abbans took a 140-foot boat mounting a Newcomen-style engine out on the Saone from Lyon. He did make it all the way back to the dock, where cheering crowds met it-just in time, before the engine's vibrations destroyed the boat.*
The great importance of Fitch's steamboat was not that it survived its inaugural trip; it was his audience, who were properly impressed with what turned out to be the steamboat's maiden voyage. One delegate, William Samuel Johnson of Connecticut, wrote on the twenty-third, "the exhibition yesterday9 gave the gentlemen present much satisfaction and will always be happy to give him every countenance and encouragement in their power which his ingenuity and industry ent.i.tles him to." Two weeks later, the Brearly Committee (named for its chairman, David Brearly of New Jersey, and also, and unfortunately, known as the "Committee of Leftovers") reported on fourteen proposals to the convention; the last one was a recommendation to "provide limited patents10 to promote science and arts." The patent clause was incorporated, without a single dissenting vote, into Article I, Section 8, paragraph 8 of the United States Const.i.tution.
It's easy to see why the American revolutionaries were so taken with the British att.i.tude toward intellectual property. In almost every relevant way, they were British. The common law was as well known on the banks of the Potomac as along the Thames. Virginians signed on to seven-year apprentices.h.i.+ps as carpenters, millwrights, and glaziers exactly as their counterparts did in Yorks.h.i.+re (sometimes an apprentices.h.i.+p would begin in the latter and conclude in the former). The eminence grise of the American Revolution, Benjamin Franklin, was not only a Fellow of the Royal Society but also one of the most prolific inventors of the entire eighteenth century; Thomas Jefferson, the revolution's intellectual soul, took enough time off from his writing and architecture to design revolving bookstands, copying machines, revolving chairs, and even a new and improved moldboard plow. If those models weren't sufficient, eighteenth-century America showed even more enthusiasm than Britain itself for the intellectual forebears of patent law. Locke was considerably more influential among the American const.i.tutionalists than he ever was to English parliamentarians, and had even drafted the first const.i.tution for the Carolina colony. And not just Locke: the Mayflower had carried a set of c.o.ke's writings from the Old World to the New, and both Jefferson and Madison had gotten their legal training from reading them. In the eighty-fourth of the Federalist Papers, Alexander Hamilton compared c.o.ke's 1628 Pet.i.tion of Right to the Magna Carta.
Fitch's influence on the future of steamboats was less enduring.* He gave up on oars fairly quickly and experimented with circular paddles and even an early propeller screw, which he used for ferry service between Philadelphia and Burlington, New Jersey, but his failures outnumbered his successes many times over. Though he pursued a national patent, and de facto monopoly, on steam-powered water travel with monomaniacal zeal for years (much of his time exhausted in disputes over priority with another inventor, James Rumsey, who used a jet of water propelled by a steam-driven pump to drive a boat on the Potomac in 1787), the final award of patent, almost three years to the day after his demonstration for the delegates in Philadelphia, was so limited in its language as to be commercially useless.
The fact that a national patent was available at all, however, was the significant thing. One reason that those const.i.tutionalists were even in Philadelphia that summer was the realization that the union originally established in 1777 under the Articles of Confederation and Perpetual Union was responsible for governing a nation that covered a territory bigger than France, Britain, Germany, and Spain combined, with fewer tools than a New England town meeting. The confederation-unable to tax its citizenry or even levy soldiers in wartime, powers that were reserved to the individual states-wasn't deriving much benefit from its size.
And while national defense was obviously a more urgent deficiency, economic issues were a close second, including the recognition that some national authority needed to promote, and protect, invention. In 1800, there were only a few more Americans living in the New World than Dutch living in the old, and we've already seen that the Netherlands, despite its great wealth, was still too small to support British-style inventing; Ma.s.sachusetts, or Virginia, wouldn't stand a chance. Inadvertently, the const.i.tution had stumbled on the fundamental issue of scale in intellectual property. The value of a bar of gold or a bushel of wheat-Romer's rivalrous property-is no greater in a large country than a small one. On the other hand, the value of a nonrivalrous patent or copyright increases in direct proportion to the number of people one can sue to prevent its theft.
Not everyone agreed, even in eighteenth-century America. Thomas Jefferson, most notably, was reflexively offended by even the slightest odor of monopoly; in a much-quoted letter sent to his friend Isaac McPherson, Jefferson wrote: If nature has made any one thing11 less susceptible than all others of exclusive property, it is the action of the thinking power called an idea, which an individual may exclusively possess as long as he keeps it to himself; but the moment it is divulged, it forces itself into the possession of everyone, and the receiver cannot dispossess himself of it. Its peculiar character, too, is that no one possesses the less, because every other possesses the whole of it. He who receives an idea from me, receives instruction himself without lessening mine; as he who lights his taper at mine, receives light without darkening me.... Inventions then cannot, in nature, be a subject of property.
Nonrivalrous property indeed; small wonder that Jefferson is regarded as the intellectual G.o.dfather of the twenty-first century's "information wants to be free" movement. Partly because of his resistance, the First Congress, which opened for business in March 1789, failed to consider a system for granting patents for nearly a year. The first American patent statute was, however, eventually pa.s.sed, and was signed by George Was.h.i.+ngton on April 10, becoming the law of the land-or of twelve-thirteenths of the land, since Rhode Island hadn't yet ratified the Const.i.tution.
The original procedure was fairly straightforward. Each patent application was sent to a committee of the United States Senate, who then referred it to the attorney general, who pa.s.sed it to the president, who signed it and returned it to the secretary of state (in 1790, Thomas Jefferson). That was changed soon enough to the newly named Commissioners for the Promotion of Useful Arts: Jefferson, Secretary of War Henry Knox, and Attorney General Edmund Randolph.
The American system was simplicity itself compared to the contemporaneous British system, which was a thing of cartoonish complexity: Step 1: Inventor prepares pet.i.tion to the Crown, including an affidavit sworn before a "Master of Chancery"
Step 2: Master sends pet.i.tion plus accompanying affidavit to the Home Office, who reads, endorses, and sends to the Attorney General and Solicitor General Step 3: The Attorney General and Solicitor General review and, if they approve, return both pet.i.tion and report to the Home Office Step 4: Home Office prepares a warrant; sends to the King Step 5: King signs, and Secretary of State countersigns, the warrant Step 6: Secretary of State sends warrant back to Attorney General and Solicitor General; they prepare a bill describing the invention, and transcribe it onto the actual letters patent (written on parchment, in order to have the force of law) Step 7: Attorney General and Solicitor General send parchment bill back to Secretary of State; King and Secretary again sign and countersign, thus making it, literally, a "King's Bill"
Step 8: Secretary of State sends the King's Bill to the Signet Office, which prepares an identical version, known as the "Signet Bill" (on parchment, of course; see Step 6) Step 9: Signet Office sends Signet Bill to the Lord Privy Seal, who prepares a Writ of Privy Seal-yes, on parchment-and sends it to the Lord Chancellor with Signet Bill and Letters Patent Step 10: Lord Chancellor "engrosses" Letters Patent on parchment with language identical to the Writ, dates it, and finally seals it The system was not only absurdly complicated but outrageously expensive. In 1792, the official cost of a patent12 was 70 for England and Wales, but "gratuities" to every secretary, official, and even doorman standing along the way typically ran another 20; the tariff including Scotland and Ireland could easily exceed 300. The cost of a U.S. patent application,13 by comparison, was fifty cents; if the patent was awarded, the recipient owed the federal government two dollars, plus another dollar for affixing the Great Seal of the United States.
Even so, the American system was a little slow getting started. The United States issued only three patents during all of 1790. The first went to Samuel Hopkins for his method of making potash; the second, "for manufacturing candles," was granted to a Boston chandler named Joseph Stacey Sampson. Both patents ended up generating far more wealth as rare doc.u.ments, sold and resold to collectors avid for the signatures of Jefferson and Was.h.i.+ngton, than they ever did for their inventors. By number three, however, the system had identified an inventor who would do as much as any man alive to put steam on the move: a Philadelphian named Oliver Evans.
EVANS WAS BORN IN 1755 in the colony of Delaware and was apprenticed at the age of sixteen to a wheelwright in Pennsylvania. By the end of the customary seven years of training and toil, he had already built his first invention, a machine tool for making the leather "cards" used for removing unwanted material from wool and cotton. One of Evans's most distinctive skills was the ability to see machines as a series of related steps, and his tool was an early, but telling, case in point: an a.s.sembly line in a box, it first bent the wire, then cut it to the proper length, and finally punched holes in precut pieces of leather, on which it mounted up to one thousand wire teeth per minute, with all the steps driven by a single rotating wheel. The hand-cranked card-making machine, for which Evans sought and received patent protection in Delaware, was an immediate success; so successful, in fact, that copies of it turned up, within months-and royalty-free-in Ma.s.sachusetts, an early reminder to the inventor of the problematic character of intellectual property.
Evans's next invention took the process of using one mechanical motion to drive a succeeding one a giant step further. Starting in 1788, he designed and built an automated mill that turned wheat into flour in a single continuous operation, ill.u.s.trating, on a much larger scale, his gift for sequential mechanics. This time an elevator-actually wooden cups affixed to belts-lifted the unmilled grains of wheat onto a conveyor belt, which, in turn, was driven by a rod with lands cut into it: effectively a horizontal screw. The belt then pushed the wheat through the millstones into a hopper where a mechanical rake alternately stirred and sifted the flour. And once again a single rotating shaft synchronized all the operations.
Of all the components of the mill, only the hopper was truly original; but the real novelty, and value, of the invention came from the way it coordinated several existing mechanisms. Evans had once again exhibited a gift for seeing the big picture, literally, if uncomfortably: "I have in my bed viewed the whole operation14 with much mental anxiety." In the event, it was the "whole operation" rather than any specific machine that earned Evans his first patents: first a fourteen-year grant in Maryland and Pennsylvania, a little later one of the same duration in Delaware, and one in New Hamps.h.i.+re to run for seven years.
His receipt of U.S. patent number 3 in 1790 generated prominent clients, and equally prominent critics. Though George Was.h.i.+ngton installed an Evans machine at his Dogue Run mill in 1791, his fellow Virginian Thomas Jefferson was nonplussed by Evans's claim of novelty: "If the bringing together under the same roof15 various useful things before known ent.i.tled him [Evans] to an exclusive use of all these ... every utensil of life might be taken for use by a patent.... I can conceive how a machine may improve the manufacturing of flour, but not how a principle abstracted from any machine can do it."
Whatever Jefferson's concerns about patents in general, and Evans's in particular, he discharged his responsibility for them with diligence, and eventually-once he conceded the success of the law in promoting invention-enthusiasm. Evans himself was certainly spurred by the financial incentives of patent owners.h.i.+p, though their promise, in his case, frequently overran their actuality. His goal had been to earn his living16 selling licenses to other millers, but he found them resistant to automation, and in a cla.s.sic pattern he spent more in legal fees suing patent infringers than he earned in licenses, despite renewing his patents nationally in 1808. By then, however, though his grist mills typically ran on waterpower, he was fully entangled in the next stage of the steam engine revolution.
In his own later recollection, Evans's interest in using steam power for transportation dated back to his apprentices.h.i.+p; in 1773, he had noticed a blacksmith's apprentice using steam as the propellant in a gun. By 1783, he was already attempting to patent a steam locomotive, though his application to the Pennsylvania legislature was rejected for lack of a working model.
Using steam to propel vehicles over land was considerably more difficult than doing so on water, for reasons of simple physics. A wheeled vehicle needs to combat both gravity and inertia, plus any number of sources of friction, particularly the wheel against the axle rod. By contrast, relatively little power is needed to propel a lot of weight on water, primarily because of the lack of any real changes in elevation, close to perfectly even terrain, and negligible friction; this is why s.h.i.+pping large amounts of freight has always been cheaper by water than by land. As a result, a separate condensing engine could drive John Fitch's steamboat. With the water supporting the engine's weight, enough power could be produced to drive the craft, though slowly: four miles an hour, a brisk walking pace.
Locomotive engines, however, needed to put out more power with less weight; to be, in short, efficient. One of Evans's great Usherian insights was that if one could dramatically increase the temperature of the steam, and therefore its pressure, the separate condenser could be dispensed with, at a huge weight savings. Another was that even though the steam, without a condenser, would simply disappear into the air once it had driven the piston, it would still use less fuel than was used up constantly reheating and recondensing. All he had to do was discover a way to increase the heat and pressure in the cylinder by an order of magnitude-but without turning it into a steam-powered hand grenade.
The key was the boiler. Low-pressure engines, from Newcomen to Watt, had boiled water in chambers whose basic design wasn't much different from that of an oversized teakettle: a pot, or "hayc.o.c.k" shape, in which the furnace was placed below the water. Despite a number of improvements, it had remained essentially unchanged ever since.
Evans's stroke of brilliance was to place his furnace inside a water-filled chamber: more surface area for the heated gas, more heat transfer to the liquid. With nowhere to go, the boiling water turned to steam whose pressure, now about fifty pounds per square inch, drove the piston, first in one direction, then in another.
In 1804, Evans applied for and received a federal patent for a high pressure "vibrating steam engine" that incorporated a "Boiler with the furnace in the center17 of the water enclosed in brick work." To Evans, like every other inventor of the steam age, heat meant fuel, so increasing the first meant adding more of the second. But he also realized "the more the steam is confined18 ... the greater will be the power obtained by the fuel. For every addition of 30 degrees of heat to the water doubles the power [and] doubling the heat of the water increases the power 100 times. Thus, the power of my engine rises in geometrical proportion, while the consumption of fuel has only an arithmetical ratio" (emphasis added). Evans had discovered how to produce the same power from a 500-pounder that Watt was getting from a two-ton monster; and as a bonus, it burned less fuel for every increase in horsepower-a huge advantage in an engine that needed not only to be mobile but also to carry its fuel with it. It was, by any definition, an act of genius.
It was not, however, a success. The value of high-pressure steam was greatest where its attractive power-to-weight ratio mattered most-steam locomotion-and America was still several decades away from railroad building. Evans was, like most inventors, forced to invest his time where he could find customers. In 1805, he built a thirty-foot-long fifteen-ton steam dredge for the Philadelphia Board of Health, which was the first steam land vehicle built in the United States; the same year, he created the first significant improvement over Watt's linkage,19 using an isosceles triangle to connect two bars at a single pivot point, a design later known as the Russell linkage.*
However, also in 1805, he was the litigant in no fewer than four different suits, brought on both philosophical and technical grounds. One result was that his income was slashed, leaving Evans, in his own words, "in poverty at the age of 50,20 with a large family of children and an amiable wife to support." Despite his many triumphs, Evans's life seemed, to him at least, a succession of unsatisfying successes and paranoia-reinforcing failures. In 1795, he had published The Young Mill-wright and Miller's Guide, which was effectively an entire millwright's education between hard covers, went through dozens of printings, and was probably the bestselling "professional" book in the young republic. But he ran out of money before completing its sequel for steam engineers, and he published it, rather petulantly, under the t.i.tle The Abortion of the Young Steam Engineer's Guide.
Fig. 7: America's first working steam "locomotive": not only the first practical high-pressure steam-powered vehicle of any sort, but the first amphibious vehicle as well, though this was largely an accident. Evans's workshop was more than a mile inland and fifteen miles from the dredging site on the Schuylkill River, so he added wheels to his paddle-driven dredge, which he had named Orukter Amphibolos, a drawing of which is in the upper right corner, and drove it proudly down Philadelphia's Market Street. Image by permission of the Library of Congress Sometimes his petulance verged on a persecution complex: "And it shall come to pa.s.s21 that the memory of those sordid and wicked wretches who opposed [my] improvements, will be execrated, by every good man, as they ought to be now...."
In 1806, however, Evans had opened the factory he called the Mars Works, where he built more than a hundred steam engines and boilers and thousands of components for mill machinery, enough that he ended
The Most Powerful Idea in the World Part 9
You're reading novel The Most Powerful Idea in the World Part 9 online at LightNovelFree.com. You can use the follow function to bookmark your favorite novel ( Only for registered users ). If you find any errors ( broken links, can't load photos, etc.. ), Please let us know so we can fix it as soon as possible. And when you start a conversation or debate about a certain topic with other people, please do not offend them just because you don't like their opinions.
The Most Powerful Idea in the World Part 9 summary
You're reading The Most Powerful Idea in the World Part 9. This novel has been translated by Updating. Author: William Rosen already has 856 views.
It's great if you read and follow any novel on our website. We promise you that we'll bring you the latest, hottest novel everyday and FREE.
LightNovelFree.com is a most smartest website for reading novel online, it can automatic resize images to fit your pc screen, even on your mobile. Experience now by using your smartphone and access to LightNovelFree.com
- Related chapter:
- The Most Powerful Idea in the World Part 8
- The Most Powerful Idea in the World Part 10
RECENTLY UPDATED NOVEL
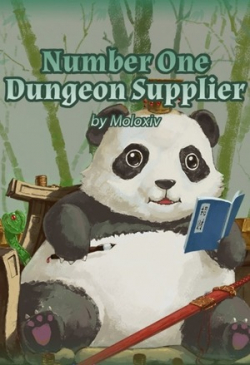
Number One Dungeon Supplier
Number One Dungeon Supplier Chapter 2221 Operation Dreaded Winter -Part 9 View : 1,261,283
I Love Destroying Worlds' Plot
I Love Destroying Worlds' Plot Chapter 1482 20.46 First Black Star - Evil Cultivators View : 508,172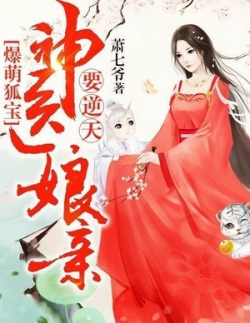
World Dominating Empress Physician
World Dominating Empress Physician Chapter 2551: Bai Yan has finally come (6) View : 665,957
Super Insane Doctor of the Goddess
Super Insane Doctor of the Goddess Chapter 1080: Who Hit You? View : 333,747