The Disappearing Spoon Part 2
You’re reading novel The Disappearing Spoon Part 2 online at LightNovelFree.com. Please use the follow button to get notification about the latest chapter next time when you visit LightNovelFree.com. Use F11 button to read novel in full-screen(PC only). Drop by anytime you want to read free – fast – latest novel. It’s great if you could leave a comment, share your opinion about the new chapters, new novel with others on the internet. We’ll do our best to bring you the finest, latest novel everyday. Enjoy!
Having no reason to refrain from using lacrimators on its own citizens (the Hague pact concerned only warfare), the French government collared a ring of Parisian bank robbers with ethyl bromoacetate in 1912. Word of this event quickly spread to France's neighbors, who were right to worry. When war broke out in August 1914, the French immediately lobbed bromine sh.e.l.ls at advancing German troops. But even Sparta two millennia before had done a better job. The sh.e.l.ls landed on a windy plain, and the gas had little effect, blowing away before the Germans realized they'd been "attacked." However, it's more accurate to say the sh.e.l.ls had little immediate immediate effect, since hysterical rumors of the gas tore through newspapers on both sides of the conflict. The Germans fanned the flames-blaming an unlucky case of carbon monoxide poisoning in their barracks on secret French asphyxiants, for instance-to justify their own chemical warfare program. effect, since hysterical rumors of the gas tore through newspapers on both sides of the conflict. The Germans fanned the flames-blaming an unlucky case of carbon monoxide poisoning in their barracks on secret French asphyxiants, for instance-to justify their own chemical warfare program.
Thanks to one man, a bald, mustached chemist who wore a pince-nez, the German gas research units soon outpaced the rest of the world's. Fritz Haber had one of the great minds in history for chemistry, and he became one of the most famous scientists in the world around 1900 when he figured out how to convert the commonest of chemicals-the nitrogen in air-into an industrial product. Although nitrogen gas can suffocate unsuspecting people, it's usually benign. In fact, it's benign almost to the point of uselessness. The one important thing nitrogen does is replenish soil: it's as crucial to plants as vitamin C is to humans. (When pitcher plants and Venus flytraps trap insects, it's the bugs' nitrogen they're after.) But even though nitrogen makes up 80 percent of air-four of every five molecules we breathe-it's surprisingly bad at topping off soil because it rarely reacts with anything and never becomes "fixed" in the soil. That combination of plent.i.tude, inept.i.tude, and importance proved a natural target for ambitious chemists.
There are many steps in the process Haber invented to "capture" nitrogen, and many chemicals appear and disappear. But basically, Haber heated nitrogen to hundreds of degrees, injected some hydrogen gas, turned up the pressure to hundreds of times greater than normal air pressure, added some crucial osmium as a catalyst, and voila: common air trans.m.u.ted into ammonia, NH3, the precursor of all fertilizers. With cheap industrial fertilizers now available, farmers no longer were limited to compost piles or dung to nourish their soil. Even by the time World War I broke out, Haber had likely saved millions from Malthusian starvation, and we can still thank him for feeding most of the world's 6.7 billion people today.*
What's lost in that summary is that Haber cared little about fertilizers, despite what he sometimes said to the contrary. He actually pursued cheap ammonia to help Germany build nitrogen explosives-the sort of fertilizer-distilled bombs that Timothy McVeigh used to blow a hole in an Oklahoma City courthouse in 1995. It's a sad truth that men like Haber pop up frequently throughout history-petty Fausts who twist scientific innovations into efficient killing devices. Haber's story is darker because he was so skilled. After World War I broke out, German military leaders, hoping to break the trench stalemate ruining their economy, recruited Haber for their gas warfare division. Though set to make a fortune from government contracts based on his ammonia patents, Haber couldn't throw away his other projects fast enough. The division was soon referred to as "the Haber office," and the military even promoted Haber, a forty-six-year-old Jewish convert to Lutheranism (it helped his career), to captain, which made him childishly proud.
His family was less impressed. Haber's uber alles stance chilled his personal relations.h.i.+ps, especially with the one person who might have redeemed him, his wife, Clara Immerwahr. She also exuded genius, becoming the first woman to earn a Ph.D. from the prestigious university in Haber's hometown, Breslau (now Wrocaw). But unlike Marie Curie, a contemporary of hers, Immerwahr never came into her own, because instead of marrying an open-minded man like Pierre Curie, she married Haber. On its face, the marriage was not a poor choice for someone with scientific ambitions, but whatever Haber's chemical brilliance, he was a flawed human being. Immerwahr, as one historian puts it, "was never out of ap.r.o.n," and she once rued to a friend about "Fritz's way of putting himself first in our home and marriage, so that a less ruthlessly a.s.sertive personality was simply destroyed." She supported Haber by translating ma.n.u.scripts into English and providing technical support on the nitrogen projects, but she refused to help on the bromine gas work.
Haber barely noticed. Dozens of other young chemists had volunteered, since Germany had fallen behind the hated French in chemical warfare, and by early 1915 the Germans had an answer to the French lacrimators. Perversely, however, the Germans tested their sh.e.l.ls on the British army, which had no gas. Fortunately, as in the first French gas attack, the wind dispersed the gas, and the British targets-bored out of their skulls in a nearby trench-had no idea they'd been attacked.
Undeterred, the German military wanted to devote even more resources to chemical warfare. But there was a problem-that pesky Hague pact, which political leaders didn't want to break (again) publicly. The solution was to interpret the pact in an ultraconscientious yet ultimately bogus way. In signing it, Germany had agreed to "abstain from the use of projectiles, the sole object of which is the diffusion of asphyxiating or deleterious gases." So to the Germans' sophisticated, legalistic reading, the pact had no jurisdiction over sh.e.l.ls that delivered shrapnel and and gas. It took some cunning engineering-the slos.h.i.+ng liquid bromine, which evaporated into gas on impact, wreaked havoc with the sh.e.l.ls' trajectory-but Germany's military-industrial-scientific complex prevailed, and a 15 cm sh.e.l.l filled with xylyl bromide, a caustic tearjerker, was ready by late 1915. The Germans called it gas. It took some cunning engineering-the slos.h.i.+ng liquid bromine, which evaporated into gas on impact, wreaked havoc with the sh.e.l.ls' trajectory-but Germany's military-industrial-scientific complex prevailed, and a 15 cm sh.e.l.l filled with xylyl bromide, a caustic tearjerker, was ready by late 1915. The Germans called it weisskreuz, weisskreuz, or "white cross." Again leaving the French alone, Germany swung its mobile gas units east, to sh.e.l.l the Russian army with eighteen thousand or "white cross." Again leaving the French alone, Germany swung its mobile gas units east, to sh.e.l.l the Russian army with eighteen thousand weisskreuze. weisskreuze. If anything, this attempt was more of a debacle than the first. The temperature in Russia was so cold the xylyl bromide froze solid. If anything, this attempt was more of a debacle than the first. The temperature in Russia was so cold the xylyl bromide froze solid.
Surveying the poor field results, Haber ditched bromine and redirected his efforts to its chemical cousin, chlorine. Chlorine sits above bromine on the periodic table and is even nastier to breathe. It's more aggressive in attacking other elements for one more electron, and because chlorine is smaller-each atom weighs less than half of a bromine atom-chlorine can attack the body's cells much more nimbly. Chlorine turns victims' skin yellow, green, and black, and gla.s.ses over their eyes with cataracts. They actually die of drowning, from the fluid buildup in their lungs. If bromine gas is a phalanx of foot soldiers clas.h.i.+ng with the mucous membranes, chlorine is a blitzkrieg tank rus.h.i.+ng by the body's defenses to tear apart the sinuses and lungs.
Because of Haber, the buffoonery of bromine warfare gave way to the ruthless chlorine phase history books memorialize today. Enemy soldiers soon had to fear the chlorine-based grunkreuz, grunkreuz, or "green cross"; the or "green cross"; the blaukreuz, blaukreuz, or "blue cross"; and the nightmarish blister agent or "blue cross"; and the nightmarish blister agent gelbkreuz, gelbkreuz, or "yellow cross," otherwise known as mustard gas. Not content with scientific contributions, Haber directed with enthusiasm the first successful gas attack in history, which left five thousand bewildered Frenchmen burned and scarred in a muddy trench near Ypres. In his spare time, Haber also coined a grotesque biological law, Haber's Rule, to quantify the relations.h.i.+p between gas concentration, exposure time, and death rate-which must have required a depressing amount of data to produce. or "yellow cross," otherwise known as mustard gas. Not content with scientific contributions, Haber directed with enthusiasm the first successful gas attack in history, which left five thousand bewildered Frenchmen burned and scarred in a muddy trench near Ypres. In his spare time, Haber also coined a grotesque biological law, Haber's Rule, to quantify the relations.h.i.+p between gas concentration, exposure time, and death rate-which must have required a depressing amount of data to produce.
Horrified by the gas projects, Clara confronted Fritz early on and demanded he cease. As usual, Fritz listened to her not at all. In fact, although he wept, quite unironically, when colleagues died during an accident in the research branch of the Haber office, after he returned from Ypres he threw a dinner party to celebrate his new weapons. Worse, Clara found out he'd come home just for the night, a stopover on his way to direct more attacks on the eastern front. Husband and wife quarreled violently, and later that night Clara walked into the family garden with Fritz's army pistol and shot herself in the chest. Though no doubt upset, Fritz did not let this inconvenience him. Without staying to make funeral arrangements, he left as planned the next morning.
Despite having the incomparable advantage of Haber, Germany ultimately lost the war to end all wars and was universally denounced as a scoundrel nation. The international reaction to Haber himself was more complicated. In 1919, before the dust (or gas) of World War I had settled, Haber won the vacant 1918 n.o.bel Prize in chemistry (the n.o.bels were suspended during the war) for his process to produce ammonia from nitrogen, even though his fertilizers hadn't protected thousands of Germans from famine during the war. A year later, he was charged with being an international war criminal for prosecuting a campaign of chemical warfare that had maimed hundreds of thousands of people and terrorized millions more-a contradictory, almost self-canceling legacy.
Things got worse. Humiliated at the huge reparations Germany had to pay to the Allies, Haber spent six futile years trying to extract dissolved gold from the oceans, so that he could pay the reparations himself. Other projects sputtered along just as uselessly, and the only thing Haber gained attention for during those years (besides trying to sell himself as a gas warfare adviser to the Soviet Union) was an insecticide. Haber had invented Zyklon A before the war, and a German chemical company tinkered with his formula after the war to produce an efficient second generation of the gas. Eventually, a new regime with a short memory took over Germany, and the n.a.z.is soon exiled Haber for his Jewish roots. He died in 1934 while traveling to England to seek refuge. Meanwhile, work on the insecticide continued. And within years the n.a.z.is were ga.s.sing millions of Jews, including relatives of Haber, with that second-generation gas-Zyklon B.
In addition to Haber's being a Jew, Germany excommunicated him because he had become pa.s.se. In parallel with its gas warfare investment, the German military had begun to exploit a different pocket of the periodic table during World War I, and it eventually decided that bludgeoning enemy combatants with two metals, molybdenum and tungsten, made more sense than scalding them with chlorine and bromine gas. Once again, then, warfare turned on simple, basic periodic table chemistry. Tungsten would go on to become the "it" metal of the Second World War, but in some ways molybdenum's story is more interesting. Almost no one knows it, but the most remote battle of World War I took place not in Siberia or against Lawrence of Arabia on the Sahara sands, but at a molybdenum mine in the Rocky Mountains of Colorado.
After its gas, Germany's most feared weapons during the war were its Big Berthas, a suite of superheavy siege guns that battered soldiers' psyches as brutally as they did the trenches of France and Belgium. The first Berthas, at forty-three tons, had to be transported in pieces by tractors to a launchpad and took two hundred men six hours to a.s.semble. The payoff was the ability to hurl a 16-inch, 2,200-pound sh.e.l.l nine miles in just seconds. Still, a big flaw hobbled the Berthas. Lofting a one-ton ma.s.s took whole kegs of gunpowder, which produced ma.s.sive amounts of heat, which in turn scorched and warped the twenty-foot steel barrels. After a few days of h.e.l.lish shooting, even if the Germans limited themselves to a few shots per hour, the gun itself was shot to h.e.l.l.
Never at a loss when providing weaponry for the fatherland, the famous Krupp armament company found a recipe for strengthening steel: spiking it with molybdenum. Molybdenum (p.r.o.nounced "mo-lib-di-num") could withstand the excessive heat because it melts at 4,750F, thousands of degrees hotter than iron, the main metal in steel. Molybdenum's atoms are larger than iron's, so they get excited more slowly, and they have 60 percent more electrons, so they absorb more heat and bind together more tightly. Plus, atoms in solids spontaneously and often disastrously rearrange themselves when temperatures change (more on this in chapter 16 chapter 16), which often results in brittle metals that crack and fail. Doping steel with molybdenum gums up the iron atoms, preventing them from sliding around. (The Germans were not the first ones to figure this out. A master sword maker in fourteenth-century j.a.pan sprinkled molybdenum into his steel and produced the island's most coveted samurai swords, whose blades never dulled or cracked. But since this j.a.panese Vulcan died with his secret, it was lost for five hundred years-proof that superior technology does not always spread and often goes extinct.) Back in the trenches, the Germans were soon blazing away at the French and British with a second generation of "moly steel" guns. But Germany soon faced another huge Bertha setback-it had no supply of molybdenum and risked running out. In fact, the only known supplier was a bankrupt, nearly abandoned mine on Bartlett Mountain in Colorado.
Before World War I, a local had laid claim to Bartlett upon discovering veins of ore that looked like lead or tin. Those metals would have been worth at least a few cents per pound, but the useless molybdenum he found cost more to mine than it fetched, so he sold his mining rights to one Otis King, a feisty five-foot-five banker from Nebraska. Always enterprising, King adopted a new extraction technique that no one had bothered to invent before and quickly liberated fifty-eight hundred pounds of pure molybdenum-which more or less ruined him. Those nearly three tons exceeded the yearly world demand for molybdenum by 50 percent, which meant King hadn't just flooded the market, he'd drowned it. Noting at least the novelty of King's attempt, the U.S. government mentioned it in a mineralogical bulletin in 1915.
Few noticed the bulletin except for a behemoth international mining company based in Frankfurt, Germany, with a U.S. branch in New York. According to one contemporary account, Metallgesellschaft had smelters, mines, refineries, and other "tentacles" all over the world. As soon as the company directors, who had close ties to Fritz Haber, read about King's molybdenum, they mobilized and ordered their top man in Colorado, Max Schott, to seize Bartlett Mountain.
Schott-a man described as having "eyes penetrating to the point of hypnosis"-sent in claim jumpers to set up stakes and hara.s.s King in court, a major drain on an already floundering mine. The more belligerent claim jumpers threatened the wives and children of miners and destroyed their camps during a winter in which the temperature dropped to twenty below. King hired a limping outlaw named Two-Gun Adams for protection, but the German agents got to King anyway, mugging him with knives and pickaxes on a mountain pa.s.s and hurling him off a sheer cliff. Only a well-placed s...o...b..nk saved his neck. As the self-described "tomboy bride" of one miner put it in her memoirs, the Germans did "everything short of downright slaughter to hinder the work of his company." King's gritty workers took to calling the unp.r.o.nounceable metal they risked their lives to dig up "Molly be d.a.m.ned."
King had a dim idea what Molly did in Germany, but he was about the only non-German in Europe or North America who did. Not until the British captured German arms in 1916 and reverse-engineered them by melting them down did the Allies discover the wundermetall, wundermetall, but the shenanigans in the Rockies continued. The United States didn't enter World War I until 1917, so it had no special reason to monitor Metallgesellschaft's subsidiary in New York, especially considering its patriotic name, American Metal. It was American Metal that Max Schott's "company" answered to, and when the government began asking questions around 1918, American Metal claimed that it legally owned the mine, since the harried Otis King had sold it to Schott for a paltry $40,000. It also admitted that, um, it just happened to s.h.i.+p all that molybdenum to Germany. The feds quickly froze Metallgesellschaft's U.S. stock and took control of Bartlett Mountain. Sadly, those efforts came too late to disable Germany's Big Berthas. As late as 1918, Germany used moly steel guns to sh.e.l.l Paris from the astonis.h.i.+ng distance of seventy-five miles. but the shenanigans in the Rockies continued. The United States didn't enter World War I until 1917, so it had no special reason to monitor Metallgesellschaft's subsidiary in New York, especially considering its patriotic name, American Metal. It was American Metal that Max Schott's "company" answered to, and when the government began asking questions around 1918, American Metal claimed that it legally owned the mine, since the harried Otis King had sold it to Schott for a paltry $40,000. It also admitted that, um, it just happened to s.h.i.+p all that molybdenum to Germany. The feds quickly froze Metallgesellschaft's U.S. stock and took control of Bartlett Mountain. Sadly, those efforts came too late to disable Germany's Big Berthas. As late as 1918, Germany used moly steel guns to sh.e.l.l Paris from the astonis.h.i.+ng distance of seventy-five miles.
The only justice was that Schott's company went bankrupt after the armistice, in March 1919, when molybdenum prices bottomed out. King returned to mining and became a millionaire by persuading Henry Ford to use moly steel in car engines. But Molly's days in warfare were over. By the time World War II rolled around, molybdenum had been superseded in steel production by the element below it on the periodic table, tungsten.
Now if molybdenum is one of the harder elements to p.r.o.nounce on the periodic table, tungsten has one of the most confounding chemical symbols, a big fat unaccountable W. It stands for wolfram, wolfram, the German name for the metal, and that "wolf" correctly portended the dark role it would play in the war. n.a.z.i Germany coveted tungsten for making machinery and armor-piercing missiles, and its l.u.s.t for the German name for the metal, and that "wolf" correctly portended the dark role it would play in the war. n.a.z.i Germany coveted tungsten for making machinery and armor-piercing missiles, and its l.u.s.t for wolfram wolfram surpa.s.sed even its l.u.s.t for looted gold, which n.a.z.i officials happily bartered for tungsten. And who were the n.a.z.is' trading partners? Not Italy and j.a.pan, the other Axis powers. Nor any of the countries German troops overran, such as Poland or Belgium. It was supposedly neutral Portugal whose tungsten fed the wolfish appet.i.te of the German surpa.s.sed even its l.u.s.t for looted gold, which n.a.z.i officials happily bartered for tungsten. And who were the n.a.z.is' trading partners? Not Italy and j.a.pan, the other Axis powers. Nor any of the countries German troops overran, such as Poland or Belgium. It was supposedly neutral Portugal whose tungsten fed the wolfish appet.i.te of the German kriegwerks. kriegwerks.
Portugal was a hard country to figure at the time. It lent the Allies a vital air base in the Azores, a group of islands in the Atlantic Ocean, and as anyone who's seen Casablanca Casablanca knows, refugees longed to escape to Lisbon, from which they could safely fly to Britain or the United States. However, the dictator of Portugal, Antonio Salazar, tolerated n.a.z.i sympathizers in his government and provided a haven for Axis spies. He also rather two-facedly s.h.i.+pped thousands of tons of tungsten to both sides during the war. Proving his worth as a former professor of economics, Salazar leveraged his country's near monopoly on the metal (90 percent of Europe's supply) into profits 1,000 percent greater than peacetime levels. This might have been defensible had Portugal had long-standing trade relations with Germany and been worried about falling into wartime poverty. But Salazar began selling tungsten to Germany in appreciable quant.i.ties only in 1941, apparently on the theory that his country's neutral status allowed him to gouge both sides equally. knows, refugees longed to escape to Lisbon, from which they could safely fly to Britain or the United States. However, the dictator of Portugal, Antonio Salazar, tolerated n.a.z.i sympathizers in his government and provided a haven for Axis spies. He also rather two-facedly s.h.i.+pped thousands of tons of tungsten to both sides during the war. Proving his worth as a former professor of economics, Salazar leveraged his country's near monopoly on the metal (90 percent of Europe's supply) into profits 1,000 percent greater than peacetime levels. This might have been defensible had Portugal had long-standing trade relations with Germany and been worried about falling into wartime poverty. But Salazar began selling tungsten to Germany in appreciable quant.i.ties only in 1941, apparently on the theory that his country's neutral status allowed him to gouge both sides equally.
The tungsten trade worked like this. Learning its lesson with molybdenum and recognizing the strategic importance of tungsten, Germany had tried to stockpile tungsten before it began erasing boundaries between itself and Poland and France. Tungsten is one of the hardest metals known, and adding it to steel made for excellent drill bits and saw heads. Plus, even modest-sized missiles tipped with tungsten-so-called kinetic energy penetrators-could take down tanks. The reason tungsten proved superior to other steel additives can be read right off the periodic table. Tungsten, situated below molybdenum, has similar properties. But with even more electrons, it doesn't melt until 6,200F. Plus, as a heavier atom than molybdenum, tungsten provides even better anchors against the iron atoms' slipping around. Remember that the nimble chlorine worked well in gas attacks. Here, in a metal, tungsten's solidity and strength proved attractive.
So attractive that the profligate n.a.z.i regime spent its entire tungsten reserve by 1941, at which point the fuhrer himself got involved. Hitler ordered his ministers to grab as much tungsten as the trains across conquered France could carry. Distressingly, far from there being a black market for this grayish metal, the whole process was entirely transparent, as one historian noted. Tungsten was s.h.i.+pped from Portugal through fascist Spain, another "neutral," and much of the gold the n.a.z.is had seized from Jews-including the gold wrenched out of the teeth of ga.s.sed Jews-was laundered by banks in Lisbon and Switzerland, still another country that took no sides. (Fifty years on, a major Lisbon bank still maintained that officials had had no idea that the forty-four tons of gold they had received were dirty, despite the swastikas stamped on many bars.) Even stalwart Britain couldn't be bothered about the tungsten that was helping to cut down its lads. Prime Minister Winston Churchill privately referred to Portugal's tungsten trade as a "misdemeanor," and lest that remark be misconstrued, he added that Salazar was "quite right" to trade tungsten with Britain's avowed enemies. Once again, however, there was a dissenter. All this naked capitalism, which benefited socialist Germany, caused apoplectic fits in the free-market United States. American officials simply couldn't grasp why Britain didn't order, or outright bully, Portugal to drop its profitable neutrality. Only after prolonged U.S. pressure did Churchill agree to help strong-arm the strongman Salazar.
Until then, Salazar (if we lay aside morality for a moment) had played the Axis and Allies brilliantly with vague promises, secret pacts, and stalling tactics that kept the tungsten trains chugging. He had increased the price of his country's one commodity from $1,100 per ton in 1940 to $20,000 in 1941, and he'd banked $170 million in three frenzied years of speculation. Only after running out of excuses did Salazar inst.i.tute a full tungsten embargo against the n.a.z.is on June 7, 1944-the day after D-Day, by which point the Allied commanders were too preoccupied (and disgusted) to punish him. I believe it was Rhett Butler in Gone with the Wind Gone with the Wind who said that fortunes can be made only during the building up or tearing down of an empire, and Salazar certainly subscribed to that theory. In the so-called wolfram war, the Portuguese dictator had the last lycanthropic laugh. who said that fortunes can be made only during the building up or tearing down of an empire, and Salazar certainly subscribed to that theory. In the so-called wolfram war, the Portuguese dictator had the last lycanthropic laugh.
Tungsten and molybdenum were only the first hints of a veritable metals revolution that would take place later in the twentieth century. Three of every four elements are metals, but beyond iron, aluminium, and a few others, most did nothing but plug holes in the periodic table before World War II. (Indeed, this book could not have been written forty years ago-there wouldn't have been enough to say.) But since about 1950, every metal has found a niche. Gadolinium is perfect for magnetic resonance imaging (MRI). Neodymium makes unprecedentedly powerful lasers. Scandium, now used as a tungstenlike additive in aluminium baseball bats and bike frames, helped the Soviet Union make lightweight helicopters in the 1980s and purportedly even topped Soviet ICBM missiles stored underground in the Arctic, to help the nukes punch through sheets of ice.
Alas, for all the technological advances made during the metals revolution, some elements continued to abet wars-and not in the remote past, but in the past decade. Fittingly, two of these elements were named after two Greek mythological characters known for great suffering. Niobe earned the ire of the G.o.ds by bragging about her seven lovely daughters and seven handsome sons-whom the easily offended Olympians soon slaughtered for her impertinence. Tantalus, Niobe's father, killed his own son and served him at a royal banquet. As punishment, Tantalus had to stand for all eternity up to his neck in a river, with a branch loaded with apples dangling above his nose. Whenever he tried to eat or drink, however, the fruit would be blown away beyond his grasp or the water would recede. Still, while elusiveness and loss tortured Tantalus and Niobe, it is actually a surfeit of their namesake elements that has decimated central Africa.
There's a good chance you have tantalum or niobium in your pocket right now. Like their periodic table neighbors, both are dense, heat-resistant, noncorrosive metals that hold a charge well-qualities that make them vital for compact cell phones. In the mid-1990s cell phone designers started demanding both metals, especially tantalum, from the world's largest supplier, the Democratic Republic of Congo, then called Zaire. Congo sits next to Rwanda in central Africa, and most of us probably remember the Rwandan butchery of the 1990s. But none of us likely remembers the day in 1996 when the ousted Rwandan government of ethnic Hutus spilled into Congo seeking refuge. At the time it seemed just to extend the Rwandan conflict a few miles west, but in retrospect it was a brush fire blown right into a decade of acc.u.mulated racial kindling. Eventually, nine countries and two hundred ethnic tribes, each with its own ancient alliances and unsettled grudges, were warring in the dense jungles.
Nonetheless, if only major armies had been involved, the Congo conflict likely would have petered out. Larger than Alaska and dense as Brazil, Congo is even less accessible than either by roads, meaning it's not ideal for waging a protracted war. Plus, poor villagers can't afford to go off and fight unless there's money at stake. Enter tantalum, niobium, and cellular technology. Now, I don't mean to impute direct blame. Clearly, cell phones didn't cause the war-hatred and grudges did. But just as clearly, the infusion of cash perpetuated the brawl. Congo has 60 percent of the world's supply of the two metals, which blend together in the ground in a mineral called coltan. Once cell phones caught on-sales rose from virtually zero in 1991 to more than a billion by 2001-the West's hunger proved as strong as Tantalus's, and coltan's price grew tenfold. People purchasing ore for cell phone makers didn't ask and didn't care where the coltan came from, and Congolese miners had no idea what the mineral was used for, knowing only that white people paid for it and that they could use the profits to support their favorite militias.
Oddly, tantalum and niobium proved so noxious because coltan was so democratic. Unlike the days when crooked Belgians ran Congo's diamond and gold mines, no conglomerates controlled coltan, and no backhoes and dump trucks were necessary to mine it. Any commoner with a shovel and a good back could dig up whole pounds of the stuff in creek beds (it looks like thick mud). In just hours, a farmer could earn twenty times what his neighbor did all year, and as profits swelled, men abandoned their farms for prospecting. This upset Congo's already shaky food supply, and people began hunting gorillas for meat, virtually wiping them out, as if they were so many buffalo. But gorilla deaths were nothing compared to the human atrocities. It's not a good thing when money pours into a country with no government. A brutal form of capitalism took over in which all things, including lives, were for sale. Huge fenced-in "camps" with enslaved prost.i.tutes sprang up, and innumerable bounties were put out for blood killings. Gruesome stories have circulated about proud victors humiliating their victims' bodies by draping themselves with entrails and dancing in celebration.
The fires burned hottest in Congo between 1998 and 2001, at which point cell phone makers realized they were funding anarchy. To their credit, they began to buy tantalum and niobium from Australia, even though it cost more, and Congo cooled down a bit. Nevertheless, despite an official truce ending the war in 2003, things never really calmed down in the eastern half of the country, near Rwanda. And lately another element, tin, has begun to fund the fighting. In 2006, the European Union outlawed lead solder in consumer goods, and most manufacturers have replaced it with tin-a metal Congo also happens to have in huge supply. Joseph Conrad once called Congo "the vilest scramble for loot that ever disfigured the history of human conscience," and there's little reason to revise that notion today.
Overall, more than five million people have died in Congo since the mid-1990s, making it the biggest waste of life since World War II. The fighting there is proof that in addition to all the uplifting moments the periodic table has inspired, it can also play on humankind's worst, most inhuman instincts.
Completing the Table... with a Bang
A supernova sowed our solar system with every natural element, and the churning of young molten planets made sure those elements were well blended in the rocky soil. But those processes alone cannot tell us everything about the distribution of elements on earth. Since the supernova, whole species of elements have gone extinct because their nuclei, their cores, were too fragile to survive in nature. This instability shocked scientists and left unaccountable holes in the periodic table-holes that, unlike in Mendeleev's time, scientists just couldn't fill, no matter how hard they searched. They eventually did fill in the table, but only after developing new fields that let them create elements on their own, and only after realizing that the fragility of some elements conceals a bright, s.h.i.+ny danger. The making of atoms and the breaking of atoms proved more intimately bound than anyone dared expect. supernova sowed our solar system with every natural element, and the churning of young molten planets made sure those elements were well blended in the rocky soil. But those processes alone cannot tell us everything about the distribution of elements on earth. Since the supernova, whole species of elements have gone extinct because their nuclei, their cores, were too fragile to survive in nature. This instability shocked scientists and left unaccountable holes in the periodic table-holes that, unlike in Mendeleev's time, scientists just couldn't fill, no matter how hard they searched. They eventually did fill in the table, but only after developing new fields that let them create elements on their own, and only after realizing that the fragility of some elements conceals a bright, s.h.i.+ny danger. The making of atoms and the breaking of atoms proved more intimately bound than anyone dared expect.
The roots of this story go back to the University of Manchester in England just before World War I. Manchester had a.s.sembled some brilliant scientists, including lab director Ernest Rutherford. Perhaps the most promising student was Henry Moseley. The son of a naturalist admired by Charles Darwin, Moseley was drawn instead to the physical sciences. He treated his lab work like a deathbed vigil, staying for fifteen-hour stretches, as if he'd never have time to finish all he wanted to do, and he subsisted on mere fruit salad and cheese. Like many gifted people, Moseley was also a pill, stiff and stuffy, and he expressed open disgust at the "scented dirtiness" of foreigners at Manchester.
But young Moseley's talent excused a lot. Although Rutherford objected to the work as a waste of time, Moseley grew enthusiastic about studying elements by blasting them with electron beams. He enlisted Darwin's grandson, a physicist, as a partner and in 1913 began to systematically probe every discovered element up to gold. As we know today, when a beam of electrons strikes an atom, the beam punches out the atom's own electrons, leaving a hole. Electrons are attracted to an atom's nucleus because electrons and protons have opposite charges, and tearing electrons away from the nucleus is a violent deed. Since nature abhors a vacuum, other electrons rush in to fill the gap, and the cras.h.i.+ng about causes them to release high-energy X-rays. Excitingly, Moseley found a mathematical relation between the wavelength of the X-rays, the number of protons an element has in its nucleus, and the element's atomic number (its spot on the periodic table).
Since Mendeleev had published his famous table in 1869, it had undergone a number of changes. Mendeleev had set his first table sideways, until someone showed him the sense in rotating it ninety degrees. Chemists continued to tinker with the table, adding columns and reshuffling elements, over the next forty years. Meanwhile, anomalies had begun to peck at people's confidence that they really understood the table. Most of the elements line up on the table in a cattle call of increasing weight. According to that criterion, nickel should precede cobalt. Yet to make the elements fit properly-so cobalt sat above cobalt-like elements and nickel above nickel-like elements-chemists had to switch their spots. No one knew why this was necessary, and it was just one of several annoying cases. To get around this problem, scientists invented the atomic number as a placeholder, which just underscored that no one knew what the atomic number actually meant.
Moseley, just twenty-five, solved the riddle by translating the question from chemistry to physics. The crucial thing to realize is that few scientists believed in the atomic nucleus at the time. Rutherford had put forward the idea of a compact, highly positive nucleus just two years earlier, and it remained unproven in 1913, too tentative for scientists to accept. Moseley's work provided the first confirmation. As Niels Bohr, another Rutherford protege, recalled, "We cannot understand it today, but [the Rutherford work] was not taken seriously.... The great change came from Moseley." That's because Moseley linked an element's place on the table to a physical characteristic, equating the positive nuclear charge with the atomic number. And he did so with an experiment that anyone could repeat. This proved the ordering of elements was not arbitrary but arose from a proper understanding of atomic anatomy. Screwy cases such as cobalt and nickel suddenly made sense, since the lighter nickel had more protons and therefore a higher positive charge and therefore had to come after cobalt. If Mendeleev and others discovered the Rubik's Cube of the elements, Moseley solved it, and after Moseley there was no more need to fudge explanations.
Furthermore, like the spectroscope, Moseley's electron gun helped tidy up the table by sorting through a confusing array of radioactive species and disproving spurious claims for new elements. Moseley also fingered just four remaining holes in the table-elements forty-three, sixty-one, seventy-two, and seventy-five. (The elements heavier than gold were too dear to obtain proper samples to experiment on in 1913. Had Moseley been able to, he would have found gaps at eighty-five, eighty-seven, and ninety-one, too.) Unfortunately, chemists and physicists mistrusted each other in this era, and some prominent chemists doubted that Moseley had come up with anything as grand as he claimed. Georges Urbain of France challenged the young Turk by bringing him an Ytterby-like blend of ambiguous rare earth elements. Urbain had labored twenty years learning rare earth chemistry, and it had taken him months of tedium to identify the four elements in his sample, so he expected to stymie if not embarra.s.s Moseley. After their initial meeting, Moseley returned to Urbain within an hour with a full and correct list.* The rare earths that had so frustrated Mendeleev were now trivial to sort out. The rare earths that had so frustrated Mendeleev were now trivial to sort out.
But they were sorted out by people other than Moseley. Although he pioneered nuclear science, as with Prometheus, the G.o.ds punished this young man whose work illuminated the darkness for later generations. When World War I broke out, Moseley enlisted in the king's army (against the army's advice) and saw action in the doomed Gallipoli campaign of 1915. One day the Turkish army rushed the British lines in phalanxes eight deep, and the battle devolved into a street fight with knives, stones, and teeth. Somewhere in that savage scrum, Moseley, age twenty-seven, fell. The futility of that war is best known through the English poets who also died on the battlefield. But one colleague spit that losing Henry Moseley by itself ensured that the war to end all wars would go down as "one of the most hideous and most irreparable crimes in history."*
The best tribute scientists could pay to Moseley was to hunt down all the missing elements he'd pointed out. Indeed, Moseley so inspired element hunters, who suddenly had a clear idea of what to search for, that element safaris became almost too popular. Scuffles soon arose over who'd first bagged hafnium, protactinium, and technetium. Other research groups filled in the gaps at elements eighty-five and eighty-seven in the late 1930s by creating elements in the lab. By 1940, only one natural element, one prize, remained undiscovered-element sixty-one.
Oddly, though, only a few research teams around the world were bothering to look for it. One team, led by an Italian physicist named Emilio Segre, tried to create an artificial sample and probably succeeded in 1942, but they gave up after a few attempts to isolate it. It wasn't until seven years later that three scientists from Oak Ridge National Laboratory in Tennessee rose at a scientific meeting in Philadelphia and announced that after sifting through some spent uranium ore, they had discovered element sixty-one. After a few hundred years of chemistry, the last hole in the periodic table had been filled.
But the announcement didn't rally much excitement. The trio announced they'd discovered element sixty-one two years before and had sat on the results because they were too preoccupied with their work on uranium-their real work. The press gave the finding correspondingly tepid coverage. In the New York Times, New York Times, the missing link shared a crowded headline with a dubious mining technique that promised a hundred uninterrupted years of oil. the missing link shared a crowded headline with a dubious mining technique that promised a hundred uninterrupted years of oil. Time Time buried the news in its conference wrap-up and pooh-poohed the element as "not good for much." buried the news in its conference wrap-up and pooh-poohed the element as "not good for much."* Then the scientists announced that they planned to name it promethium. Elements discovered earlier in the century had been given boastful or at least explanatory names, but promethium-after the t.i.tan in Greek mythology who stole fire, gave it to humankind, and was tortured by having a vulture dine on his liver-evoked something stern and grim, even guilty. Then the scientists announced that they planned to name it promethium. Elements discovered earlier in the century had been given boastful or at least explanatory names, but promethium-after the t.i.tan in Greek mythology who stole fire, gave it to humankind, and was tortured by having a vulture dine on his liver-evoked something stern and grim, even guilty.
So what happened between Moseley's time and the discovery of element sixty-one? Why had hunting for elements gone from work so important that a colleague had called Moseley's death an irreparable crime to work worth barely a few lines of newsprint? Sure, promethium was useless, but scientists, of all people, cheer impractical discoveries, and the completion of the periodic table was epochal, the culmination of millions of man-hours. Nor had people simply gotten fatigued with seeking new elements-that pursuit caused sparring between American and Soviet scientists through much of the cold war. Instead, the nature and enormity of nuclear science had changed. People had seen things, seen things, and a mid-range element like promethium could no longer rouse them like the heavy elements plutonium and uranium, not to mention their famous offspring, the atomic bomb. and a mid-range element like promethium could no longer rouse them like the heavy elements plutonium and uranium, not to mention their famous offspring, the atomic bomb.
One morning in 1939, a young physicist at the University of California at Berkeley settled into a pneumatic barber's chair in the student union for a haircut. Who knows the topic of conversation that day-maybe that son of a b.i.t.c.h Hitler or whether the Yankees would win their fourth straight World Series. Regardless, Luis Alvarez (not yet famous for his dinosaur extinction theory) was chatting and leafing through the San Francisco Chronicle San Francisco Chronicle when he ran across a wire service item about experiments by Otto Hahn in Germany, on fission-the splitting of the uranium atom. Alvarez halted his barber "mid-snip," as a friend recalled, tore off his smock, and sprinted up the road to his laboratory, where he scooped up a Geiger counter and made a beeline for some irradiated uranium. His hair still only half-cut, he summoned everyone within shouting distance to come see what Hahn had discovered. when he ran across a wire service item about experiments by Otto Hahn in Germany, on fission-the splitting of the uranium atom. Alvarez halted his barber "mid-snip," as a friend recalled, tore off his smock, and sprinted up the road to his laboratory, where he scooped up a Geiger counter and made a beeline for some irradiated uranium. His hair still only half-cut, he summoned everyone within shouting distance to come see what Hahn had discovered.
Beyond being amusing, Alvarez's dash symbolizes the state of nuclear science at the time. Scientists had been making steady if slow progress in understanding how the cores of atoms work, little snippets of knowledge here and there-and then, with one discovery, they found themselves on a mad tear.
Moseley had given atomic and nuclear science legitimate footing, and loads of talent had poured into those fields in the 1920s. Nevertheless, gains had proved more difficult than expected. Part of the confusion was, indirectly, Moseley's fault. His work had proved that isotopes such as lead-204 and lead-206 could have the same net positive charge yet have different atomic weights. In a world that knew only about protons and electrons, this left scientists floundering with unwieldy ideas about positive protons in the nucleus that gobbled up negative electrons Pac-Man style.* In addition, to comprehend how subatomic particles behave, scientists had to devise a whole new mathematical tool, quantum mechanics, and it took years to figure out how to apply it to even simple, isolated hydrogen atoms. In addition, to comprehend how subatomic particles behave, scientists had to devise a whole new mathematical tool, quantum mechanics, and it took years to figure out how to apply it to even simple, isolated hydrogen atoms.
Meanwhile, scientists were also developing the related field of radioactivity, the study of how nuclei fall apart. Any old atom can shed or steal electrons, but luminaries such as Marie Curie and Ernest Rutherford realized that some rare elements could alter their nuclei, too, by blowing off atomic shrapnel. Rutherford especially helped cla.s.sify all the shrapnel into just a few common types, which he named using the Greek alphabet, calling them alpha, beta, or gamma decay. Gamma decay is the simplest and deadliest-it occurs when the nucleus emits concentrated X-rays and is today the stuff of nuclear nightmares. The other types of radioactivity involve the conversion of one element to another, a tantalizing process in the 1920s. But each element goes radioactive in a characteristic way, so the deep, underlying features of alpha and beta decay baffled scientists, who were growing increasingly frustrated about the nature of isotopes as well. The Pac-Man model was failing, and a few daredevils suggested that the only way to deal with the proliferation of new isotopes was to sc.r.a.p the periodic table.
The giant collective forehead slap-the "Of course!" moment-took place in 1932, when James Chadwick, yet another of Rutherford's students, discovered the neutral neutron, which adds weight without charge. Coupled with Moseley's insights about the atomic number, atoms (at least lone, isolated atoms) suddenly made sense. The neutron meant that lead-204 and lead-206 could still both be lead-could still have the same positive nuclear charge and sit in the same box on the periodic table-even if they had different atomic weights. The nature of radioactivity suddenly made sense, too. Beta decay was understood as the conversion of neutrons to protons or vice versa-and it's because the proton number changes that beta decay converts an atom into a different element. Alpha decay also converts elements and is the most dramatic change on a nuclear level-two neutrons and two protons are shorn away.
Over the next few years, the neutron became more than a theoretical tool. For one thing, it supplied a fantastic way to probe atomic innards, because scientists could shoot a neutron at atoms without it being electrically repulsed, as charged projectiles were. Neutrons also helped scientists induce a new type of radioactivity. Elements, especially lighter elements, try to maintain a rough one-to-one ratio of neutrons to protons. If an atom has too many neutrons, it splits itself, releasing energy and excess neutrons in the process. If nearby atoms absorb those neutrons, they become unstable and spit out more neutrons, a cascade known as a chain reaction. A physicist named Leo Szilard dreamed up the idea of a nuclear chain reaction circa 1933 while standing at a London stoplight one morning. He patented it in 1934 and tried (but failed) to produce a chain reaction in a few light elements as early as 1936.
But notice the dates here. Just as the basic understanding of electrons, protons, and neutrons fell into place, the old-world political order was disintegrating. By the time Alvarez read about uranium fission in his barber's smock, Europe was doomed.
The genteel old world of element hunting died at the same time. With their new model of atomic innards, scientists began to see that the few undiscovered elements on the periodic table were undiscovered because they were intrinsically unstable. Even if they had existed in abundance on the early earth, they had long since disintegrated. This conveniently explained the holes in the periodic table, but the work proved its own undoing. Probing unstable elements soon led scientists to stumble onto nuclear fission and neutron chain reactions. And as soon as they understood that atoms could be split-understood both the scientific and political implications of that fact-collecting new elements for display seemed like an amateur's hobby, like the fusty, shoot-and-stuff biology of the 1800s compared with molecular biology today. Which is why, with a world war and the possibility of atomic bombs staring at them in 1939, no scientists bothered tracking promethium down until a decade later.
No matter how keyed up scientists got about the possibility of fission bombs, however, a lot of work still separated the theory from the reality. It's hard to remember today, but nuclear bombs were considered a long shot at best, especially by military experts. As usual, those military leaders were eager to enlist scientists in World War II, and the scientists dutifully exacerbated the war's gruesomeness through technology such as better steel. But the war would not have ended with two mushroom clouds had the U.S. government, instead of just demanding bigger, faster weapons now, now, summoned the political will to invest billions in a hitherto pure and impractical field: subatomic science. And even then, figuring out how to divide atoms in a controlled manner proved so far beyond the science of the day that the Manhattan Project had to adopt a whole new research strategy to succeed-the Monte Carlo method, which rewired people's conceptions of what "doing science" meant. summoned the political will to invest billions in a hitherto pure and impractical field: subatomic science. And even then, figuring out how to divide atoms in a controlled manner proved so far beyond the science of the day that the Manhattan Project had to adopt a whole new research strategy to succeed-the Monte Carlo method, which rewired people's conceptions of what "doing science" meant.
As noted, quantum mechanics worked fine for isolated atoms, and by 1940 scientists knew that absorbing a neutron made an atom queasy, which made it explode and possibly release more neutrons. Following the path of one given neutron was easy, no harder than following a caroming billiard ball. But starting a chain reaction required coordinating billions of billions of neutrons, all of them traveling at different speeds in every direction. This made hash of scientists' built-for-one theoretical apparatus. At the same time, uranium and plutonium were expensive and dangerous, so detailed experimental work was out of the question.
Yet Manhattan Project scientists had orders to figure out exactly how much plutonium and uranium they needed to create a bomb: too little and the bomb would fizzle out. Too much and the bomb would blow up just fine, but at the cost of prolonging the war by months, since both elements were monstrously complicated to purify (or in plutonium's case, synthesize, then purify). So, just to get by, some pragmatic scientists decided to abandon both traditional approaches, theory and experiment, and pioneer a third path.
To start, they picked a random speed for a neutron bouncing around in a pile of plutonium (or uranium). They also picked a random direction for it and more random numbers for other parameters, such as the amount of plutonium available, the chance the neutron would escape the plutonium before being absorbed, even the geometry and shape of the plutonium pile. Note that selecting specific numbers meant that scientists were conceding the universality of each calculation, since the results applied to only a few neutrons in one of many designs. Theoretical scientists hate hate giving up universally applicable results, but they had no other choice. giving up universally applicable results, but they had no other choice.
At this point, rooms full of young women with pencils (many of them scientists' wives, who'd been hired to help out because they were crus.h.i.+ngly bored in Los Alamos) would get a sheet with the random numbers and begin to calculate (sometimes without knowing what it all meant) how the neutron collided with a plutonium atom; whether it was gobbled up; how many new neutrons if any were released in the process; how many neutrons those in turn released; and so on. Each of the hundreds of women did one narrow calculation in an a.s.sembly line, and scientists aggregated the results. Historian George Dyson described this process as building bombs "numerically, neutron by neutron, nanosecond by nanosecond... [a method] of statistical approximation whereby a random sampling of events... is followed through a series of representative slices in time, answering the otherwise incalculable question of whether a configuration would go thermonuclear." neutron by neutron, nanosecond by nanosecond... [a method] of statistical approximation whereby a random sampling of events... is followed through a series of representative slices in time, answering the otherwise incalculable question of whether a configuration would go thermonuclear."*
Sometimes the theoretical pile did go nuclear, and this was counted as a success. When each calculation was finished, the women would start over with different numbers. Then do it again. And again. And yet again. Rosie the Riveter may have become the iconic symbol of empowered female employment during the war, but the Manhattan Project would have gone nowhere without these women hand-crunching long tables of data. They became known by the neologism "computers."
But why was this approach so different? Basically, scientists equated each computation with an experiment and collected only virtual data for the plutonium and uranium bombs. They abandoned the meticulous and mutually corrective interplay of theory and lab work and adopted methods one historian described unflatteringly as "dislocated... a simulated reality that borrowed from both experimental and theoretical domains, fused these borrowings together, and used the resulting amalgam to stake out a netherland at once nowhere and everywhere on the usual methodological map."*
Of course, such calculations were only as good as scientists' initial equations, but here they got lucky. Particles on the quantum level are governed by statistical laws, and quantum mechanics, for all its bizarre, counterintuitive features, is the single most accurate scientific theory ever devised. Plus, the sheer number of calculations scientists pushed through during the Manhattan Project gave them great confidence-confidence that was proved justified after the successful Trinity test in New Mexico in mid-1945. The swift and flawless detonation of a uranium bomb over Hiros.h.i.+ma and a plutonium bomb over Nagasaki a few weeks later also testified to the accuracy of this unconventional, calculation-based approach to science.
After the isolated camaraderie of the Manhattan Project ended, scientists scattered back to their homes to reflect on what they'd done (some proudly, some not). Many gladly forgot about their time served in the calculation wards. Some, though, were riveted by what they'd learned, including one Stanislaw Ulam. Ulam, a Polish refugee who'd pa.s.sed hours in New Mexico playing card games, was playing solitaire one day in 1946 when he began wondering about the odds of winning any randomly dealt hand. The one thing Ulam loved more than cards was futile calculation, so he began filling pages with probabilistic equations. The problem soon ballooned to such complexity that Ulam smartly gave up. He decided it was better to play a hundred hands and tabulate what percentage of the time he won. Easy enough.
The neurons of most people, even most scientists, wouldn't have made the connection, but in the middle of his century of solitaire, Ulam recognized that he was using the same basic approach as scientists had used in the bomb-building "experiments" in Los Alamos. (The connections are abstract, but the order and layout of the cards were like the random inputs, and the "calculation" was playing the hand.) Discussions soon followed with his calculation-loving friend John von Neumann, another European refugee and Manhattan Project veteran. Ulam and von Neumann realized just how powerful the method might be if they could universalize it and apply it to other situations with mult.i.tudes of random variables. In those situations, instead of trying to take into account every complication, every b.u.t.terfly flapping its wings, they would simply define the problem, pick random inputs, and "plug and chug." Unlike an experiment, the results were not certain. But with enough calculations, they could be pretty darn sure of the probabilities.
In a serendipitous coincidence, Ulam and von Neumann knew the American engineers developing the first electronic computers, such as the ENIAC in Philadelphia. The Manhattan Project "computers" had eventually employed a mechanical punch card system for calculations, but the tireless ENIAC showed more promise for the tedious iterations Ulam and von Neumann envisioned. Historically, the science of probability has its roots in aristocratic casinos, and it's unclear where the nickname for Ulam and von Neumann's approach came from. But Ulam liked to brag that he named it in memory of an uncle who often borrowed money to gamble on the "well-known generator of random integers (between zero and thirty-six) in the Mediterranean princ.i.p.ality."
Regardless, Monte Carlo science caught on quickly. It cut down on expensive experiments, and the need for high-quality Monte Carlo simulators drove the early development of computers, pus.h.i.+ng them to become faster and more efficient. Symbiotically, the advent of cheap computing meant that Monte Carlostyle experiments, simulations, and models began to take over branches of chemistry, astronomy, and physics, not to mention engineering and stock market a.n.a.lysis. Today, just two generations on, the Monte Carlo method (in various forms) so dominates some fields that many young scientists don't realize how thoroughly they've departed from traditional theoretical or experimental science. Overall, an expedient, a temporary measure-using plutonium and uranium atoms like an abacus to compute nuclear chain reactions-has become an irreplaceable feature of the scientific process. It not only conquered science; it settled down, a.s.similated, and intermarried with other methods.
In 1949, however, that transformation lay in the future. In those early days, Ulam's Monte Carlo method mostly pushed through the next generation of nuclear weapons. Von Neumann, Ulam, and their ilk would show up at the gymnasium-sized rooms where computers were set up and mysteriously ask if they could run a few programs, starting at 12:00 a.m. and running through the night. The weapons they developed during those dead hours were the "supers," multistage devices a thousand times more powerful than standard A-bombs. Supers used plutonium and uranium to ignite stellar-style fusion in extraheavy liquid hydrogen, a complicated process that never would have moved beyond secret military reports and into missile silos without digital computation. As historian George Dyson neatly summarized the technological history of that decade, "Computers led to bombs, and bombs led to computers."
After a great struggle to find the proper design for a super, scientists. .h.i.t upon a dandy in 1952. The obliteration of the Eniwetok atoll in the Pacific Ocean during a test of a super that year showed once again the ruthless brilliance of the Monte Carlo method. Nevertheless, bomb scientists already had something even worse than the supers in the pipeline.
Atomic bombs can get you two ways. A madman who just wants lots of people dead and lots of buildings flattened can stick with a conventional, one-stage fission bomb. It's easier to build, and the big flash-bang should satisfy his need for spectacle, as should aftereffects such as spontaneous tornadoes and the silhouettes of victims seared onto brick walls. But if the madman has patience and wants to do something insidious, if he wants to p.i.s.s in every well and sow the ground with salt, he'll detonate a cobalt-60 dirty bomb.
Whereas conventional nuclear bombs kill with heat, dirty bombs kill with gamma radiation-malignant X-rays. Gamma rays result from frantic radioactive events, and in addition to burning people frightfully, they dig down into bone marrow and scramble the chromosomes in white blood cells. The cells either die outright, grow cancerous, or grow without constraint and, like humans with gigantism, end up deformed and unable to fight infections. All nuclear bombs release some radiation, but with dirty bombs, radiation is the whole point.
Even endemic leukemia isn't ambitious by some bombs' standards. Another European refugee who worked on the Manhattan Project, Leo Szilard-the physicist who, to his regret, invented the idea of a self-sustaining nuclear chain reaction around 1933-calculated in 1950 as a wiser, more sober man that sprinkling a tenth of an ounce of cobalt-60 on every square mile of earth would pollute it with enough gamma rays to wipe out the human race, a nuclear version of the cloud that helped kill the dinosaurs. His device consisted of a multistage warhead surrounded by a jacket of cobalt-59. A fission reaction in plutonium would kick off a fusion reaction in hydrogen, and once the reaction started, obviously, the cobalt jacket and everything else would be obliterated. But not before something happened on the atomic level. Down there, the cobalt atoms would absorb neutrons from the fission and fusion, a step called salting. The salting would convert stable cobalt-59 into unsteady cobalt-60, which would then float down like ash.
Lots of other elements emit gamma rays, but there's something special about cobalt. Regular A-bombs can be waited out in underground shelters, since their fallout will vomit up gamma rays immediately and be rendered harmless. Hiros.h.i.+ma and Nagasaki were more or less habitable within days of the 1945 explosions. Other elements absorb extra neutrons like alcoholics do another shot at the bar-they'll get sick someday but not for eons. In that case, after the initial blast, radiation levels would never climb too high.
Cobalt bombs fall devilishly between those extremes, a rare case in which the golden mean is the worst. Cobalt-60 atoms would settle into the ground like tiny land mine
The Disappearing Spoon Part 2
You're reading novel The Disappearing Spoon Part 2 online at LightNovelFree.com. You can use the follow function to bookmark your favorite novel ( Only for registered users ). If you find any errors ( broken links, can't load photos, etc.. ), Please let us know so we can fix it as soon as possible. And when you start a conversation or debate about a certain topic with other people, please do not offend them just because you don't like their opinions.
The Disappearing Spoon Part 2 summary
You're reading The Disappearing Spoon Part 2. This novel has been translated by Updating. Author: Sam Kean already has 1046 views.
It's great if you read and follow any novel on our website. We promise you that we'll bring you the latest, hottest novel everyday and FREE.
LightNovelFree.com is a most smartest website for reading novel online, it can automatic resize images to fit your pc screen, even on your mobile. Experience now by using your smartphone and access to LightNovelFree.com
- Related chapter:
- The Disappearing Spoon Part 1
- The Disappearing Spoon Part 3
RECENTLY UPDATED NOVEL

Emperor’s Domination
Emperor’s Domination Chapter 6242: You'll Be Copying Me Later View : 17,978,427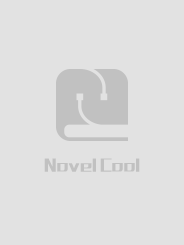
Reborn : Space Intelligent Woman
Reborn : Space Intelligent Woman Chapter 2136: Thought They Are Intruders View : 1,302,771
Warning : Providence the Beauty is Driven to Villainy
Warning : Providence the Beauty is Driven to Villainy Chapter 1269: She is guilty of the same crime as love (16)_1 View : 319,218
Warlock Apprentice
Warlock Apprentice Chapter 1098: Section 1099 The Trace of Canaan View : 1,068,910
The Yun Family's Ninth Child Is An Imp!
The Yun Family's Ninth Child Is An Imp! Chapter 6290 View : 2,205,322
Fairy Tail: Collapsing Stars
Fairy Tail: Collapsing Stars Fairy Tail Collapsing Stars Chapter 315 View : 136,100
Lord of Mysteries 2: Circle of Inevitability
Lord of Mysteries 2: Circle of Inevitability Chapter 1129 A Chance Encounter View : 618,656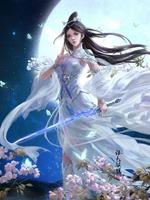