A History of Science Volume III Part 14
You’re reading novel A History of Science Volume III Part 14 online at LightNovelFree.com. Please use the follow button to get notification about the latest chapter next time when you visit LightNovelFree.com. Use F11 button to read novel in full-screen(PC only). Drop by anytime you want to read free – fast – latest novel. It’s great if you could leave a comment, share your opinion about the new chapters, new novel with others on the internet. We’ll do our best to bring you the finest, latest novel everyday. Enjoy!
Planche discovered that sheets of lead immersed in dilute sulphuric acid were very satisfactory for the production of polarization effects. He constructed a battery of sheets of lead immersed in sulphuric acid, and, after charging these for several hours from the cells of an ordinary Bunsen battery, was able to get currents of great strength and considerable duration. This battery, however, from its construction of lead, was necessarily heavy and c.u.mbersome. Faure improved it somewhat by coating the lead plates with red-lead, thus increasing the capacity of the cell. Faure's invention gave a fresh impetus to inventors, and shortly after the market was filled with storage batteries of various kinds, most of them modifications of Planche's or Faure's. The ardor of enthusiastic inventors soon flagged, however, for all these storage batteries proved of little practical account in the end, as compared with other known methods of generating power.
Three methods of generating electricity are in general use: static or frictional electricity is generated by "plate" or "static" machines; galvanic, generated by batteries based on Volta's discovery; and induced, or faradic, generated either by chemical or mechanical action.
There is still another kind, thermo-electricity, that may be generated in a most simple manner. In 1821 Seebecle, of Berlin, discovered that when a circuit was formed of two wires of different metals, if there be a difference in temperature at the juncture of these two metals an electrical current will be established. In this way heat may be transmitted directly into the energy of the current without the interposition of the steam-engine. Batteries constructed in this way are of low resistance, however, although by arranging several of them in "series," currents of considerable strength can be generated. As yet, however, they are of little practical importance.
About the middle of the century Clerk-Maxwell advanced the idea that light waves were really electro-magnetic waves. If this were true and light proved to be simply one form of electrical energy, then the same would be true of radiant heat. Maxwell advanced this theory, but failed to substantiate it by experimental confirmation. But Dr. Heinrich Hertz, a few years later, by a series of experiments, demonstrated the correctness of Maxwell's surmises. What are now called "Hertzian waves"
are waves apparently identical with light waves, but of much lower pitch or period. In his experiments Hertz showed that, under proper conditions, electric sparks between polished b.a.l.l.s were attended by ether waves of the same nature as those of light, but of a pitch of several millions of vibrations per second. These waves could be dealt with as if they were light waves--reflected, refracted, and polarized.
These are the waves that are utilized in wireless telegraphy.
ROENTGEN RAYS, OR X-RAYS
In December of 1895 word came out of Germany of a scientific discovery that startled the world. It came first as a rumor, little credited; then as a p.r.o.nounced report; at last as a demonstration. It told of a new manifestation of energy, in virtue of which the interior of opaque objects is made visible to human eyes. One had only to look into a tube containing a screen of a certain composition, and directed towards a peculiar electrical apparatus, to acquire clairvoyant vision more wonderful than the discredited second-sight of the medium. Coins within a purse, nails driven into wood, spectacles within a leather case, became clearly visible when subjected to the influence of this magic tube; and when a human hand was held before the tube, its bones stood revealed in weird simplicity, as if the living, palpitating flesh about them were but the shadowy substance of a ghost.
Not only could the human eye see these astounding revelations, but the impartial evidence of inanimate chemicals could be brought forward to prove that the mind harbored no illusion. The photographic film recorded the things that the eye might see, and ghostly pictures galore soon gave a quietus to the doubts of the most sceptical. Within a month of the announcement of Professor Roentgen's experiments comment upon the "X-ray" and the "new photography" had become a part of the current gossip of all Christendom.
It is hardly necessary to say that such a revolutionary thing as the discovery of a process whereby opaque objects became transparent, or translucent, was not achieved at a single bound with no intermediate discoveries. In 1859 the German physicist Julius Plucker (1801-1868) noticed that when there was an electrical discharge through an exhausted tube at a low pressure, on the surrounding walls of the tube near the negative pole, or cathode, appeared a greenish phosph.o.r.escence. This discovery was soon being investigated by a number of other scientists, among others. .h.i.ttorf, Goldstein, and Professor (now Sir William) Crookes. The explanations given of this phenomenon by Professor Crookes concern us here more particularly, inasmuch as his views did not accord exactly with those held by the other two scientists, and as his researches were more directly concerned in the discovery of the Roentgen rays. He held that the heat and phosph.o.r.escence produced in a low-pressure tube were caused by streams of particles, projected from the cathode with great velocity, striking the sides of the gla.s.s tube.
The composition of the gla.s.s seemed to enter into this phosph.o.r.escence also, for while lead gla.s.s produced blue phosph.o.r.escence, soda gla.s.s produced a yellowish green. The composition of the gla.s.s seemed to be changed by a long-continued pelting of these particles, the phosph.o.r.escence after a time losing its initial brilliancy, caused by the gla.s.s becoming "tired," as Professor Crookes said. Thus when some opaque substance, such as iron, is placed between the cathode and the sides of the gla.s.s tube so that it casts a shadow in a certain spot on the gla.s.s for some little time, it is found on removing the opaque substance or changing its position that the area of gla.s.s at first covered by the shadow now responded to the rays in a different manner from the surrounding gla.s.s.
The peculiar ray's, now known as the cathode rays, not only cast a shadow, but are deflected by a magnet, so that the position of the phosph.o.r.escence on the sides of the tube may be altered by the proximity of a powerful magnet. From this it would seem that the rays are composed of particles charged with negative electricity, and Professor J. J.
Thomson has modified the experiment of Perrin to show that negative electricity is actually a.s.sociated with the rays. There is reason for believing, therefore, that the cathode rays are rapidly moving charges of negative electricity. It is possible, also, to determine the velocity at which these particles are moving by measuring the deflection produced by the magnetic field.
From the fact that opaque substances cast a shadow in these rays it was thought at first that all solids were absolutely opaque to them. Hertz, however, discovered that a small amount of phosph.o.r.escence occurred on the gla.s.s even when such opaque substances as gold-leaf or aluminium foil were interposed between the cathode and the sides of the tube.
Shortly afterwards Lenard discovered that the cathode rays can be made to pa.s.s from the inside of a discharge tube to the outside air. For convenience these rays outside the tube have since been known as "Lenard rays."
In the closing days of December, 1895, Professor Wilhelm Konrad Roentgen, of Wurzburg, announced that he had made the discovery of the remarkable effect arising from the cathode rays to which reference was made above. He found that if a plate covered with a phosph.o.r.escent substance is placed near a discharge tube exhausted so highly that the cathode rays produced a green phosph.o.r.escence, this plate is made to glow in a peculiar manner. The rays producing this glow were not the cathode rays, although apparently arising from them, and are what have since been called the Roentgen rays, or X-rays.
Roentgen found that a shadow is thrown upon the screen by substances held between it and the exhausted tube, the character of the shadow depending upon the density of the substance. Thus metals are almost completely opaque to the rays; such substances as bone much less so, and ordinary flesh hardly so at all. If a coin were held in the hand that had been interposed between the tube and the screen the picture formed showed the coin as a black shadow; and the bones of the hand, while casting a distinct shadow, showed distinctly lighter; while the soft tissues produced scarcely any shadow at all. The value of such a discovery was obvious from the first; and was still further enhanced by the discovery made shortly that, photographic plates are affected by the rays, thus making it possible to make permanent photographic records of pictures through what we know as opaque substances.
What adds materially to the practical value of Roentgen's discovery is the fact that the apparatus for producing the X-rays is now so simple and relatively inexpensive that it is within the reach even of amateur scientists. It consists essentially of an induction coil attached either to cells or a street-current plug for generating the electricity, a focus tube, and a phosph.o.r.escence screen. These focus tubes are made in various shapes, but perhaps the most popular are in the form of a gla.s.s globe, not unlike an ordinary small-sized water-bottle, this tube being closed and exhausted, and having the two poles (anode and cathode) sealed into the gla.s.s walls, but protruding at either end for attachment to the conducting wires from the induction coil. This tube may be mounted on a stand at a height convenient for manipulation.
The phosph.o.r.escence screen is usually a plate covered with some platino-cyanide and mounted in the end of a box of convenient size, the opposite end of which is so shaped that it fits the contour of the face, shutting out the light and allowing the eyes of the observer to focalize on the screen at the end. For making observations the operator has simply to turn on the current of electricity and apply the screen to his eyes, pointing it towards the glowing tube, when the shadow of any substance interposed between the tube and the screen will appear upon the phosph.o.r.escence plate.
The wonderful shadow pictures produced on the phosph.o.r.escence screen, or the photographic plate, would seem to come from some peculiar form of light, but the exact nature of these rays is still an open question.
Whether the Roentgen rays are really a form of light--that is, a form of "electro-magnetic disturbance propagated through ether," is not fully determined. Numerous experiments have been undertaken to determine this, but as yet no proof has been found that the rays are a form of light, although there appears to be nothing in their properties inconsistent with their being so. For the moment most investigators are content to admit that the term X-ray virtually begs the question as to the intimate nature of the form of energy involved.
VIII. THE CONSERVATION OF ENERGY
As we have seen, it was in 1831 that Faraday opened up the field of magneto-electricity. Reversing the experiments of his predecessors, who had found that electric currents may generate magnetism, he showed that magnets have power under certain circ.u.mstances to generate electricity; he proved, indeed, the interconvertibility of electricity and magnetism.
Then he showed that all bodies are more or less subject to the influence of magnetism, and that even light may be affected by magnetism as to its phenomena of polarization. He satisfied himself completely of the true ident.i.ty of all the various forms of electricity, and of the convertibility of electricity and chemical action. Thus he linked together light, chemical affinity, magnetism, and electricity. And, moreover, he knew full well that no one of these can be produced in indefinite supply from another. "Nowhere," he says, "is there a pure creation or production of power without a corresponding exhaustion of something to supply it."
When Faraday wrote those words in 1840 he was treading on the very heels of a greater generalization than any which he actually formulated; nay, he had it fairly within his reach. He saw a great truth without fully realizing its import; it was left for others, approaching the same truth along another path, to point out its full significance.
The great generalization which Faraday so narrowly missed is the truth which since then has become familiar as the doctrine of the conservation of energy--the law that in transforming energy from one condition to another we can never secure more than an equivalent quant.i.ty; that, in short, "to create or annihilate energy is as impossible as to create or annihilate matter; and that all the phenomena of the material universe consist in transformations of energy alone." Some philosophers think this the greatest generalization ever conceived by the mind of man. Be that as it may, it is surely one of the great intellectual landmarks of the nineteenth century. It stands apart, so stupendous and so far-reaching in its implications that the generation which first saw the law developed could little appreciate it; only now, through the vista of half a century, do we begin to see it in its true proportions.
A vast generalization such as this is never a mushroom growth, nor does it usually spring full grown from the mind of any single man. Always a number of minds are very near a truth before any one mind fully grasps it. Pre-eminently true is this of the doctrine of the conservation of energy. Not Faraday alone, but half a dozen different men had an inkling of it before it gained full expression; indeed, every man who advocated the undulatory theory of light and heat was verging towards the goal.
The doctrine of Young and Fresnel was as a highway leading surely on to the wide plain of conservation. The phenomena of electro-magnetism furnished another such highway. But there was yet another road which led just as surely and even more readily to the same goal. This was the road furnished by the phenomena of heat, and the men who travelled it were destined to outstrip their fellow-workers; though, as we have seen, wayfarers on other roads were within hailing distance when the leaders pa.s.sed the mark.
In order to do even approximate justice to the men who entered into the great achievement, we must recall that just at the close of the eighteenth century Count Rumford and Humphry Davy independently showed that labor may be transformed into heat; and correctly interpreted this fact as meaning the transformation of molar into molecular motion. We can hardly doubt that each of these men of genius realized--vaguely, at any rate--that there must be a close correspondence between the amount of the molar and the molecular motions; hence that each of them was in sight of the law of the mechanical equivalent of heat. But neither of them quite grasped or explicitly stated what each must vaguely have seen; and for just a quarter of a century no one else even came abreast their line of thought, let alone pa.s.sing it.
But then, in 1824, a French philosopher, Sadi Carnot, caught step with the great Englishmen, and took a long leap ahead by explicitly stating his belief that a definite quant.i.ty of work could be transformed into a definite quant.i.ty of heat, no more, no less. Carnot did not, indeed, reach the clear view of his predecessors as to the nature of heat, for he still thought it a form of "imponderable" fluid; but he reasoned none the less clearly as to its mutual convertibility with mechanical work.
But important as his conclusions seem now that we look back upon them with clearer vision, they made no impression whatever upon his contemporaries. Carnot's work in this line was an isolated phenomenon of historical interest, but it did not enter into the scheme of the completed narrative in any such way as did the work of Rumford and Davy.
The man who really took up the broken thread where Rumford and Davy had dropped it, and wove it into a completed texture, came upon the scene in 1840. His home was in Manchester, England; his occupation that of a manufacturer. He was a friend and pupil of the great Dr. Dalton.
His name was James Prescott Joule. When posterity has done its final juggling with the names of the nineteenth century, it is not unlikely that the name of this Manchester philosopher will be a household word, like the names of Aristotle, Copernicus, and Newton.
For Joule's work it was, done in the fifth decade of the century, which demonstrated beyond all cavil that there is a precise and absolute equivalence between mechanical work and heat; that whatever the form of manifestation of molar motion, it can generate a definite and measurable amount of heat, and no more. Joule found, for example, that at the sea-level in Manchester a pound weight falling through seven hundred and seventy-two feet could generate enough heat to raise the temperature of a pound of water one degree Fahrenheit. There was nothing haphazard, nothing accidental, about this; it bore the stamp of unalterable law.
And Joule himself saw, what others in time were made to see, that this truth is merely a particular case within a more general law. If heat cannot be in any sense created, but only made manifest as a transformation of another kind of motion, then must not the same thing be true of all those other forms of "force"--light, electricity, magnetism--which had been shown to be so closely a.s.sociated, so mutually convertible, with heat? All a.n.a.logy seemed to urge the truth of this inference; all experiment tended to confirm it. The law of the mechanical equivalent of heat then became the main corner-stone of the greater law of the conservation of energy.
But while this citation is fresh in mind, we must turn our attention with all haste to a country across the Channel--to Denmark, in short--and learn that even as Joule experimented with the transformation of heat, a philosopher of Copenhagen, Colding by name, had hit upon the same idea, and carried it far towards a demonstration. And then, without pausing, we must s.h.i.+ft yet again, this time to Germany, and consider the work of three other men, who independently were on the track of the same truth, and two of whom, it must be admitted, reached it earlier than either Joule or Colding, if neither brought it to quite so clear a demonstration. The names of these three Germans are Mohr, Mayer, and Helmholtz. Their share in establis.h.i.+ng the great doctrine of conservation must now claim our attention.
As to Karl Friedrich Mohr, it may be said that his statement of the doctrine preceded that of any of his fellows, yet that otherwise it was perhaps least important. In 1837 this thoughtful German had grasped the main truth, and given it expression in an article published in the Zeitschrift fur Physik, etc. But the article attracted no attention whatever, even from Mohr's own countrymen. Still, Mohr's t.i.tle to rank as one who independently conceived the great truth, and perhaps conceived it before any other man in the world saw it as clearly, even though he did not demonstrate its validity, is not to be disputed.
It was just five years later, in 1842, that Dr. Julius Robert Mayer, practising physician in the little German town of Heilbronn, published a paper in Liebig's Annalen on "The Forces of Inorganic Nature," in which not merely the mechanical theory of heat, but the entire doctrine of the conservation of energy, is explicitly if briefly stated. Two years earlier Dr. Mayer, while surgeon to a Dutch India vessel cruising in the tropics, had observed that the venous blood of a patient seemed redder than venous blood usually is observed to be in temperate climates. He pondered over this seemingly insignificant fact, and at last reached the conclusion that the cause must be the lesser amount of oxidation required to keep up the body temperature in the tropics. Led by this reflection to consider the body as a machine dependent on outside forces for its capacity to act, he pa.s.sed on into a novel realm of thought, which brought him at last to independent discovery of the mechanical theory of heat, and to the first full and comprehensive appreciation of the great law of conservation. Blood-letting, the modern physician holds, was a practice of very doubtful benefit, as a rule, to the subject; but once, at least, it led to marvellous results. No straw is go small that it may not point the receptive mind of genius to new and wonderful truths.
MAYER'S PAPER OF 1842
The paper in which Mayer first gave expression to his revolutionary ideas bore the t.i.tle of "The Forces of Inorganic Nature," and was published in 1842. It is one of the gems of scientific literature, and fortunately it is not too long to be quoted in its entirety. Seldom if ever was a great revolutionary doctrine expounded in briefer compa.s.s:
"What are we to understand by 'forces'? and how are different forces related to each other? The term force conveys for the most part the idea of something unknown, unsearchable, and hypothetical; while the term matter, on the other hand, implies the possession, by the object in question, of such definite properties as weight and extension. An attempt, therefore, to render the idea of force equally exact with that of matter is one which should be welcomed by all those who desire to have their views of nature clear and unenc.u.mbered by hypothesis.
"Forces are causes; and accordingly we may make full application in relation to them of the principle causa aequat effectum. If the cause c has the effect e, then c = e; if, in its turn, e is the cause of a second effect of f, we have e = f, and so on: c = e = f... = c. In a series of causes and effects, a term or a part of a term can never, as is apparent from the nature of an equation, become equal to nothing.
This first property of all causes we call their indestructibility.
"If the given cause c has produced an effect e equal to itself, it has in that very act ceased to be--c has become e. If, after the production of e, c still remained in the whole or in part, there must be still further effects corresponding to this remaining cause: the total effect of c would thus be > e, which would be contrary to the supposition c = e. Accordingly, since c becomes e, and e becomes f, etc., we must regard these various magnitudes as different forms under which one and the same object makes its appearance. This capability of a.s.suming various forms is the second essential property of all causes. Taking both properties together, we may say, causes an INDESTRUCTIBLE quant.i.tatively, and quant.i.tatively CONVERTIBLE objects.
"There occur in nature two causes which apparently never pa.s.s one into the other," said Mayer. "The first cla.s.s consists of such causes as possess the properties of weight and impenetrability. These are kinds of matter. The other cla.s.s is composed of causes which are wanting in the properties just mentioned--namely, forces, called also imponderables, from the negative property that has been indicated. Forces are therefore INDESTRUCTIBLE, CONVERTIBLE, IMPONDERABLE OBJECTS.
"As an example of causes and effects, take matter: explosive gas, H + O, and water, HO, are related to each other as cause and effect; therefore H + O = HO. But if H + O becomes HO, heat, cal., makes its appearance as well as water; this heat must likewise have a cause, x, and we have therefore H + O + X = HO + cal. It might be asked, however, whether H + O is really = HO, and x = cal., and not perhaps H + O = cal., and x = HO, whence the above equation could equally be deduced; and so in many other cases. The phlogistic chemists recognized the equation between cal. and x, or phlogiston as they called it, and in so doing made a great step in advance; but they involved themselves again in a system of mistakes by putting x in place of O. In this way they obtained H = HO + x.
"Chemistry teaches us that matter, as a cause, has matter for its effect; but we may say with equal justification that to force as a cause corresponds force as effect. Since c = e, and e = c, it is natural to call one term of an equation a force, and the other an effect of force, or phenomenon, and to attach different notions to the expression force and phenomenon. In brief, then, if the cause is matter, the effect is matter; if the cause is a force, the effect is also a force.
"The cause that brings about the raising of a weight is a force. The effect of the raised weight is, therefore, also a force; or, expressed in a more general form, SEPARATION IN s.p.a.cE OF PONDERABLE OBJECTS IS A FORCE; and since this force causes the fall of bodies, we call it FALLING FORCE. Falling force and fall, or, still more generally, falling force and motion, are forces related to each other as cause and effect--forces convertible into each other--two different forms of one and the same object. For example, a weight resting on the ground is not a force: it is neither the cause of motion nor of the lifting of another weight. It becomes so, however, in proportion as it is raised above the ground. The cause--that is, the distance between a weight and the earth, and the effect, or the quant.i.ty of motion produced, bear to each other, as shown by mechanics, a constant relation.
"Gravity being regarded as the cause of the falling of bodies, a gravitating force is spoken of; and thus the ideas of PROPERTY and of FORCE are confounded with each other. Precisely that which is the essential attribute of every force--that is, the UNION of indestructibility with convertibility--is wanting in every property: between a property and a force, between gravity and motion, it is therefore impossible to establish the equation required for a rightly conceived causal relation. If gravity be called a force, a cause is supposed which produces effects without itself diminis.h.i.+ng, and incorrect conceptions of the causal connections of things are thereby fostered. In order that a body may fall, it is just as necessary that it be lifted up as that it should be heavy or possess gravity. The fall of bodies, therefore, ought not to be ascribed to their gravity alone. The problem of mechanics is to develop the equations which subsist between falling force and motion, motion and falling force, and between different motions. Here is a case in point: The magnitude of the falling force v is directly proportional (the earth's radius being a.s.sumed--oo) to the magnitude of the ma.s.s m, and the height d, to which it is raised--that is, v = md. If the height d = l, to which the ma.s.s m is raised, is transformed into the final velocity c = l of this ma.s.s, we have also v = mc; but from the known relations existing between d and c, it results that, for other values of d or of c, the measure of the force v is mc squared; accordingly v = md = mcsquared. The law of the conservation of vis viva is thus found to be based on the general law of the indestructibility of causes.
"In many cases we see motion cease without having caused another motion or the lifting of a weight. But a force once in existence cannot be annihilated--it can only change its form. And the question therefore arises, what other forms is force, which we have become acquainted with as falling force and motion, capable of a.s.suming? Experience alone can lead us to a conclusion on this point. That we may experiment to advantage, we must select implements which, besides causing a real cessation of motion, are as little as possible altered by the objects to be examined. For example, if we rub together two metal plates, we see motion disappear, and heat, on the other hand, make its appearance, and there remains to be determined only whether MOTION is the cause of heat.
In order to reach a decision on this point, we must discuss the question whether, in the numberless cases in which the expenditure of motion is accompanied by the appearance of heat, the motion has not some other effect than the production of heat, and the heat some other cause than the motion.
"A serious attempt to ascertain the effects of ceasing motion has never been made. Without wis.h.i.+ng to exclude a priori the hypothesis which it may be possible to establish, therefore, we observe only that, as a rule, this effect cannot be supposed to be an alteration in the state of aggregation of the moved (that is, rubbing, etc.) bodies. If we a.s.sume that a certain quant.i.ty of motion v is expended in the conversion of a rubbing substance m into n, we must then have m + v - n, and n = m + v; and when n is reconverted into m, v must appear again in some form or other.
"By the friction of two metallic plates continued for a very long time, we can gradually cause the cessation of an immense quant.i.ty of movement; but would it ever occur to us to look for even the smallest trace of the force which has disappeared in the metallic dust that we could collect, and to try to regain it thence? We repeat, the motion cannot have been annihilated; and contrary, or positive and negative, motions cannot be regarded as = o any more than contrary motions can come out of nothing, or a weight can raise itself.
"Without the recognition of a causal relation between motion and heat, it is just as difficult to explain the production of heat as it is to give any account of the motion that disappears. The heat cannot be derived from the diminution of the volume of the rubbing substances.
It is well known that two pieces of ice may be melted by rubbing them together in vacuo; but let any one try to convert ice into water by pressure, however enormous. The author has found that water undergoes a rise of temperature when shaken violently. The water so heated (from twelve to thirteen degrees centigrade) has a greater bulk after being shaken than it had before. Whence now comes this quant.i.ty of heat, which by repeated shaking may be called into existence in the same apparatus as often as we please? The vibratory hypothesis of heat is an approach towards the doctrine of heat being the effect of motion, but it does not favor the admission of this causal relation in its full generality. It rather lays the chief stress on restless oscillations.
A History of Science Volume III Part 14
You're reading novel A History of Science Volume III Part 14 online at LightNovelFree.com. You can use the follow function to bookmark your favorite novel ( Only for registered users ). If you find any errors ( broken links, can't load photos, etc.. ), Please let us know so we can fix it as soon as possible. And when you start a conversation or debate about a certain topic with other people, please do not offend them just because you don't like their opinions.
A History of Science Volume III Part 14 summary
You're reading A History of Science Volume III Part 14. This novel has been translated by Updating. Author: Henry Smith Williams already has 693 views.
It's great if you read and follow any novel on our website. We promise you that we'll bring you the latest, hottest novel everyday and FREE.
LightNovelFree.com is a most smartest website for reading novel online, it can automatic resize images to fit your pc screen, even on your mobile. Experience now by using your smartphone and access to LightNovelFree.com
RECENTLY UPDATED NOVEL

My Girlfriend is a Zombie
My Girlfriend is a Zombie Chapter 844: Xiao Bai in the Grass View : 2,302,128
Alchemy Emperor Of The Divine Dao
Alchemy Emperor Of The Divine Dao Chapter 4357 Another Deal View : 13,711,374
Cultivating In Secret Beside A Demoness
Cultivating In Secret Beside A Demoness Chapter 1318: Senior, I'm Tired of Standing (1) View : 510,550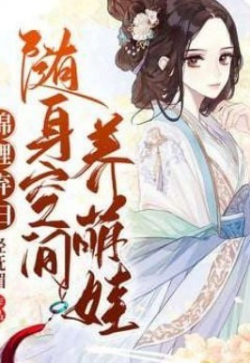
Raising My Children With My Personal Spatial Ability
Raising My Children With My Personal Spatial Ability Chapter 1805 I Actually Don't Want to... View : 1,248,991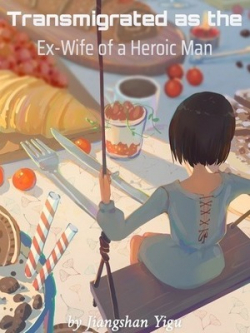
Transmigrated as the Ex-Wife of a Heroic Man
Transmigrated as the Ex-Wife of a Heroic Man Chapter 1313: Refreshing One's Worldview View : 578,989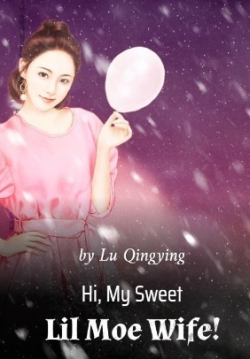