A History of Science Volume V Part 2
You’re reading novel A History of Science Volume V Part 2 online at LightNovelFree.com. Please use the follow button to get notification about the latest chapter next time when you visit LightNovelFree.com. Use F11 button to read novel in full-screen(PC only). Drop by anytime you want to read free – fast – latest novel. It’s great if you could leave a comment, share your opinion about the new chapters, new novel with others on the internet. We’ll do our best to bring you the finest, latest novel everyday. Enjoy!
These six refractory gases now became a target for the experiments of a host of workers in all parts of the world. The resources of mechanical ingenuity of the time were exhausted in the effort to produce low temperatures on the one hand and high pressures on the other. Thus Andrews, in England, using the bath of solid carbonic acid and ether which Thilorier had discovered, and which produces a degree of cold of--80 Centigrade, applied a pressure of five hundred atmospheres, or nearly four tons to the square inch, without producing any change of state. Natterer increased this pressure to two thousand seven hundred atmospheres, or twenty-one tons to the square inch, with the same negative results. The result of Andrews' experiments in particular was the final proof of what Cagniard de la Tour had early suspected and Faraday had firmly believed, that pressure alone, regardless of temperature, is not sufficient to reduce a gas to the liquid state. In other words, the fact of a so-called "critical temperature," varying for different substances, above which a given substance is always a gas, regardless of pressure, was definitively discovered. It became clear, then, that before the resistant gases would be liquefied means of reaching extremely low temperatures must be discovered. And for this, what was needed was not so much new principles as elaborate and costly machinery for the application of a principle long familiar--the principle, namely, that an evaporating liquid reduces the temperature of its immediate surroundings, including its own substance.
Ingenious means of applying this principle, in connection with the means previously employed, were developed independently by Pictet in Geneva and Cailletet in Paris, and a little later by the Cracow professors Wroblewski and Olzewski, also working independently. Pictet, working on a commercial scale, employed a series of liquefied gases to gain lower and lower temperatures by successive stages. Evaporating sulphurous acid liquefied carbonic acid, and this in evaporating brought oxygen under pressure to near its liquefaction point; and, the pressure being suddenly released (a method employed in Faraday's earliest experiments), the rapid expansion of the compressed oxygen liquefies a portion of its substance. This result was obtained in 1877 by Pictet and Cailletet almost simultaneously. Cailletet had also liquefied the newly discovered acetylene gas. Five years later Wroblewski liquefied marsh gas, and the following year nitrogen; while carbonic oxide and nitrous oxide yielded to Olzewski in 1884. Thus forty years of effort had been required to conquer five of Faraday's refractory gases, and the sixth, hydrogen, still remains resistant. Hydrogen had, indeed, been seen to a.s.sume the form of visible vapor, but it had not been reduced to the so-called static state--that is, the droplets had not been collected in an appreciable quant.i.ty, as water is collected in a cup. Until this should be done, the final problem of the liquefaction of hydrogen could not be regarded as satisfactorily solved.
More than another decade was required to make this final step in the completion, of Faraday's work. And, oddly enough, yet very fittingly, it was reserved for Faraday's successor in the chair at the Royal Inst.i.tution to effect this culmination. Since 1884 Professor Dewar's work has made the Royal Inst.i.tution again the centre of low-temperature research. By means of improved machinery and of ingenious devices for s.h.i.+elding the substance operated on from the accession of heat, to which reference will be made more in detail presently, Professor Dewar was able to liquefy the gas fluorine, recently isolated by Moussan, and the recently discovered gas helium in 1897. And in May, 1898, he was able to announce that hydrogen also had yielded, and for the first time in the history of science that* elusive substance, hitherto "permanently"
gaseous, was held as a tangible liquid in a cuplike receptacle; and this closing scene of the long struggle was enacted in the same laboratory in which Faraday performed the first liquefaction experiment with chlorine just three-quarters of a century before.
It must be noted, however, that this final stage in the liquefaction struggle was not effected through the use of the principle of evaporating liquids which has just been referred to, but by the application of a quite different principle and its elaboration into a perfectly novel method. This principle is the one established long ago by Joule and Thomson (Lord Kelvin), that compressed gases when allowed to expand freely are lowered in temperature. In this well-known principle the means was at hand greatly to simplify and improve the method of liquefaction of gases, only for a long time no one recognized the fact. Finally, however, the idea had occurred to two men almost simultaneously and quite independently. One of these was Professor Linde, the well-known German experimenter with refrigeration processes; the other, Dr. William Hampson, a young English physician. Each of these men conceived the idea--and ultimately elaborated it in practice--of acc.u.mulating the cooling effect of an expanding gas by allowing the expansion to take place through a small orifice into a chamber in which the coil containing the compressed gas was held. In Dr. Hampson's words:
"The method consists in directing all the gas immediately after its expansion over the coils which contain the compressed gas that is on its way to the expansion-point. The cold developed by expansion in the first expanded gas is thus communicated to the oncoming compressed gas, which consequently expands from, and therefore to, a lower temperature than the preceding portion. It communicates in the same way its own intensified cold to the succeeding portion of compressed gas, which, in its turn, is made colder, both before and after expansion, than any that had gone before. This intensification of cooling goes on until the expansion-temperature is far lower than it was at starting; and if the apparatus be well arranged the effect is so powerful that even the smaller amount of cooling due to the free expansion of gas through a throttle-valve, though p.r.o.nounced by Siemens and Coleman incapable of being utilized, may be made to liquefy air without using other refrigerants."
So well is this principle carried out in Dr. Hamp-son's apparatus for liquefying air that compressed air pa.s.sing into the coil at ordinary temperature without other means of refrigeration begins to liquefy in about six minutes--a result that seems almost miraculous when it is understood that the essential mechanism by which this is brought about is contained in a cylinder only eighteen inches long and seven inches in diameter.
As has been said, it was by adopting this principle of self-intensive refrigeration that Professor Dewar was able to liquefy hydrogen. More recently the same result has been attained through use of the same principle by Professor Ramsay and Dr. Travers at University College, London, who are to be credited also with first publis.h.i.+ng a detailed account of the various stages of the process. It appears that the use of the self-intensification principle alone is not sufficient with hydrogen as it is with the less volatile gases, including air, for the reason that at all ordinary temperatures hydrogen does not cool in expanding, but actually becomes warmer. It is only after the compressed hydrogen has been cooled by immersion in refrigerating media of very low temperature that this gas becomes amenable to the law of cooling on expansion. In the apparatus used at University College the coil of compressed hydrogen is pa.s.sed successively through (1) a jar containing alcohol and solid carbonic acid at a temperature of--80 Centigrade; (2) a chamber containing liquid air at atmospheric pressure, and (3) liquid air boiling in a vacuum bringing the temperature to perhaps 2050 Centigrade before entering the Hampson coil, in which expansion and the self-intensive refrigeration lead to actual liquefaction. With this apparatus Dr. Travers succeeded in producing an abundant quant.i.ty of liquid hydrogen for use in the experiments on the new gases that were first discovered in the same laboratory through the experiments on liquid air--gases about which I shall have something more to say in another chapter.
PRINCIPLES AND EXPERIMENTS
At first blush it seems a very marvellous thing, this liquefaction of substances that under all ordinary conditions are gaseous. It is certainly a little startling to have a cup of clear, water-like liquid offered one, with the a.s.surance that it is nothing but air; still more so to have the same air presented in the form of a white "avalanche snow." In a certain sense it is marvellous, because the mechanical difficulties that have been overcome in reducing the air to these unusual conditions are great. Yet, in another and broader view, there is nothing more wonderful about liquid air than about liquid water, or liquid mercury, or liquid iron. Long before air was actually liquefied, it was perfectly understood by men of science that under certain conditions it could be liquefied just as surely as water, mercury, iron, and every other substance could be brought to a similar state. This being known, and the principles involved understood, had there been nothing more involved than the bare effort to realize these conditions all the recent low-temperature work would have been mere scientific child's-play, and liquid air would be but a toy of science. But in point of fact there are many other things than this involved; new principles were being searched for and found in the course of the application of the old ones; new light was being thrown into many dark corners; new fields of research, some of them as yet barely entered, were being thrown open to the investigator; new applications of energy, of vast importance not merely in pure science but in commercial life as well, were being made available. That is why the low-temperature work must be regarded as one of the most important scientific accomplishments of our century.
At the very outset it was this work in large measure which gave the final answer to the long-mooted question as to the nature of heat, demonstrating the correctness of Count Rumford's view that heat is only a condition not itself a substance. Since about the middle of the century this view, known as the mechanical theory of heat, has been the constant guide of the physicists in all their experiments, and any one who would understand the low-temperature phenomena must keep this conception of the nature of heat clearly and constantly in mind. To understand the theory, one must think of all matter as composed of minute isolated particles or molecules, which are always in motion--vibrating, if you will. He must mentally magnify and visualize these particles till he sees them quivering before him, like tuning-forks held in the hand. Remember, then, that, like the tuning-fork, each molecule would, if left to itself, quiver less and less violently, until it ran down altogether, but that the motion thus lessening is not really lost. It is sent out in the form of ether waves, which can set up like motion in any other particles which they reach, be they near or remote; or it is transmitted as a direct push--a kick, if you will--to any other particle with which the molecule comes in physical contact.
But note now, further, that our molecule, while incessantly giving out its energy of motion in ether waves and in direct pushes, is at the same time just as ceaslessly receiving motion from the ether waves made by other atoms, and by the return push of the molecules against which it pushes. In a word, then, every molecule of matter is at once a centre for the distribution of motion (sending out impulses which affect, sooner or later, every other atom of matter in the universe), and, from the other point of view, also a centre for the reception of motion from every direction and from every other particle of matter in the universe.
Whether any given molecule will on the whole gain motion or lose it depends clearly on the simple mechanical principles of give and take.
From equally familiar mechanical principles, it is clear that our vibrating molecule, in virtue of its vibrations, is elastic, tending to be thrown back from every other molecule with which it comes in contact, just as a vibrating tuning-fork kicks itself away from anything it touches. And of course the vigor of the recoil will depend upon the vigor of the vibration and the previous movements. But since these movements const.i.tute temperature, this is another way of saying that the higher the temperature of a body the more its molecules will tend to spring asunder, such separation in the aggregate const.i.tuting expansion of the ma.s.s as a whole. Thus the familiar fact of expansion of a body under increased temperature is explained.
But now, since all molecules are vibrating, and so tending to separate, it is clear that no unconfined ma.s.s of molecules would long remain in contiguity unless some counter influence tended to draw them together.
Such a counter influence in fact exists, and is termed the "force" of cohesion. This force is a veritable gravitation influence, drawing every molecule towards every other molecule. Possibly it is identical with gravitation. It seems subject to some law of decreasing in power with the square of the distance; or, at any rate, it clearly becomes less potent as the distance through which it operates increases.
Now, between this force of cohesion which tends to draw the molecules together, and the heat vibrations which tend to throw the molecules farther asunder, there seems to be an incessant battle. If cohesion prevails, the molecules are held for the time into a relatively fixed system, which we term the solid state. If the two forces about balance each other, the molecules move among themselves more freely but maintain an average distance, and we term the condition the liquid state. But if the heat impulse preponderates, the molecules (unless restrained from without) fly farther and farther asunder, moving so actively that when they collide the recoil is too great to be checked by cohesion, and this condition we term the gaseous state.
Now after this statement, it is clear that what the low-temperature worker does when he would liquefy a gas is to become the champion of the force of cohesion. He cannot directly aid it, for so far as is known it is an unalterable quant.i.ty, like gravitation. But he can accomplish the same thing indirectly by weakening the power of the rival force. Thus, if he encloses a portion of gas in a cylinder and drives a piston down against it, he is virtually aiding cohesion by forcing the molecules closer together, so that the hold of cohesion, acting through a less distance, is stronger. What he accomplishes here is not all gain, however, for the bounding molecules, thus jammed together, come in collision with one another more and more frequently, and thus their average activity of vibration is increased and not diminished; in other words, the temperature of the gas has risen in virtue of the compression. Compression alone, then, will not avail to enable cohesion to win the battle.
But the physicist has another resource. He may place the cylinder of gas in a cold medium, so that the heat vibrations sent into it will be less vigorous than those it sends out. That is a blow the molecule cannot withstand. It is quite impotent to cease sending out the impulses however little comes in return; hence the aggregate motion becomes less and less active, until finally the molecule is moving so sluggishly that when it collides with its fellow cohesion is able to hold it there.
Cohesion, then, has won the battle, and the gas has become a liquid.
Such, stated in terms of the mechanical theory of heat, is what is brought to pa.s.s when a gas is liquefied in the laboratory of the physicist. It remains only to note that different chemical substances show the widest diversity as to the exact point of temperature at which this balance of the expansive and cohesive tendencies is affected, but that the point, under uniform conditions of pressure, is always the same for the same substance. This diversity has to do pretty clearly with the size of the individual molecules involved; but its exact explanation is not yet forthcoming, and, except in a general way, the physicist would not be able to predict the "critical temperature" of any new gas presented to him. But once this has been determined by experiment, he always knows just what to expect of any given substance. He knows, for example, that in a mixture of gases hydrogen would still remain gaseous after all the others had a.s.sumed the liquid state, and most of them the solid state as well.
These mechanical conceptions well in mind, it is clear that what the would-be liquefier of gases has all along sought to attain is merely the insulation of the portion of matter with which he worked against the access of heat-impulse from its environment. It is clear that were any texture known which would permit a heat-impulse to pa.s.s through it in one direction only, nothing more would be necessary than to place a portion of gas in such a receptacle of this substance, so faced as to permit egress but not entrance of the heat, and the gas thus enclosed, were it hydrogen itself, would very soon become liquid and solid, through spontaneous giving off of its energy, without any manipulation whatever. Contrariwise, were the faces of the receptacle reversed, a piece of iron placed within it would be made red-hot and melted though the receptacle were kept packed in salt and ice and no heat applied except such as came from this freezing mixture. One could cook a beefsteak with a cake of ice had he but such a material as this with which to make his stove. Not even Rumford or our modern Edward Atkinson ever dreamed of such economy of fuel as that.
But, unfortunately, no such substance as this is known, nor, indeed, any substance that will fully prevent the pa.s.sage of heat-impulses in either direction. Hence one of the greatest tasks of the experimenters has been to find a receptacle that would insulate a cooled substance even partially from the incessant bombardment of heat-impulses from without.
It is obvious that unless such an insulating receptacle could be provided none of the more resistent gases, such as oxygen, could be long kept liquid, even when once brought to that condition, since an environment of requisite frigidity could not practicably be provided.
But now another phase of the problem presents itself to the experimenter. Oxygen has a.s.sumed the quiescent liquid state, to be sure, but in so doing it has fallen below the temperature of its cooling medium; hence it is now receiving from that medium more energy of vibration than it gives, and unless this is prevented very soon its particles will again have power to kick themselves apart and resume the gaseous state. Something, then, must be done to insulate the liquefied gas, else it will retain the liquid state for too short a time to be much experimented with. How might such insulation be accomplished?
The most successful attack upon this important problem has been made by Professor Dewar. He invented a receptacle for holding liquefied gases which, while not fulfilling the ideal conditions referred to above, yet accomplishes a very remarkable degree of heat insulation. In consists of a gla.s.s vessel with double walls, the s.p.a.ce between which is rendered a vacuum of the highest practicable degree. This vacuum, containing practically no particles of matter, cannot, of course, convey heat-impulses to or from the matter in the receptacle with any degree of rapidity. Thus one of the two possible means of heat transfer is shut off and a degree of insulation afforded the liquefied substance. But of course the other channel, ether radiation, remains. Even this may be blocked to a large extent, however, by leaving a trace of mercury vapor in the vacuum s.p.a.ce, which will be deposited as a fine mirror on the inner surface of the chamber. This mirror serves as an admirable reflector of the heat-rays that traverse the vacuum, sending more than half of them back again. So, by the combined action of vacuum and mirror, the amount of heat that can penetrate to the interior of the receptacle is reduced to about one-thirtieth of what would enter an ordinary vessel. In other words, a quant.i.ty of liquefied gas which would evaporate in one minute from an ordinary vessel will last half an hour in one of Professor Dewar's best vacuum vessels. Thus in one of these vessels a quant.i.ty of liquefied air, for example, can be kept for a considerable time in an atmosphere at ordinary temperature, and will only volatilize at the surface, like water under the same conditions, though of course more rapidly; whereas the same liquid in an ordinary vessel would boil briskly away, like water over a fire. Only, be it remembered, the air in "boiling" is at a temperature of about one hundred and eighty degrees below zero, so that it would instantly freeze almost any substance placed into it. A portion of alcohol poured on its surface will be changed quickly into a globule of ice, which will rattle about the sides of the vessel like a marble. That is not what one ordinarily thinks of as a "boiling" temperature.
If the vacuum vessel containing a liquefied gas be kept in a cold medium, and particularly if two vacuum tubes be placed together, so that no exposed surface of liquid remains, a portion of liquefied air, for example, may be kept almost indefinitely. Thus it becomes possible to utilize the liquefied gas for experimental investigation of the properties of matter at low temperatures that otherwise would be quite impracticable. Great numbers of such experiments have been performed in the past decade or so by all the workers with low temperatures already mentioned, and by various others, including, fittingly enough, the holder of the Rumford professors.h.i.+p of experimental physics at Harvard, Professor Trowbridge. The work of Professor Dewar has perhaps been the most comprehensive and varied, but the researches of Pictet, Wroblewski, and Olzewski have also been important, and it is not always possible to apportion credit for the various discoveries accurately, since the authorities themselves are in unfortunate disagreement in several questions of priority. But in any event, such questions of exact priority have no great interest for any one but the persons directly involved. We may quite disregard them here, confining attention to the results themselves, which are full of interest.
The questions investigated have to do with the physical properties, such as electrical conductivity, magnetic condition, light-absorption, cohesion, and chemical affinities of matter at excessively low temperatures. It is found that in all these regards most substances are profoundly modified when excessively cooled. Thus if a piece of any pure metal is placed in an electric circuit and plunged into liquid air, its resistance to the pa.s.sage of the electricity steadily decreases as the metal cools, until at the temperature of the liquid it is very trifling indeed. The conclusion seems to be justified that if the metal could be still further cooled until it reached the theoretical "absolute zero,"
or absolutely heatless condition, the electrical resistance would also be nil. So it appears that the heat vibrations of the molecules of a pure metal interfere with the electrical current. The thought suggests itself that this may be because the ether waves set up by the vibrating molecules conflict with the ether strain which is regarded by some theorists as const.i.tuting the electrical "current." But this simple explanation falters before further experiments which show, paradoxically enough, that the electrical resistance of carbon exactly reverses what has just been said of pure metals, becoming greater and greater as the carbon is cooled. If an hypothesis were invented to cover this case there would still remain a puzzle in the fact that alloys of metals do not act at all like the pure metals themselves, the electrical resistance of such alloys being, for the most part, unaffected by changed temperature. On the whole, then, the facts of electrical conduction at low temperatures are quite beyond the reach of present explanation. They must await a fuller knowledge of molecular conditions in general than is at present available--a knowledge to which the low-temperature work itself seems one of the surest channels.
Even further beyond the reach of present explanation are the facts as to magnetic conditions at low temperatures. Even as to the facts themselves different experimenters have differed somewhat, but the final conclusion of Professor Dewar is that, after a period of fluctuation, the power of a magnet repeatedly subjected to a liquid-air bath becomes permanently increased. Various substances not markedly magnetic at ordinary temperatures become so when cooled. Among these, as Professor Dewar discovered, is liquid oxygen itself. Thus if a portion of liquid air be further cooled until it a.s.sumes a semi-solid condition, the oxygen may be drawn from the ma.s.s by a magnet, leaving a pure nitrogen jelly. These facts are curious enough, and full of suggestion, but like all other questions having to do with magnetism, they hold for the present generation the double fascination of insoluble mystery. To be sure, one may readily enough suggest that if magnetism be really a whirl in the ether, this whirl is apparently interfered with by the waves of radiant heat; or, again, that magnetism is presumably due to molecular motions which are apparently interfered with by another kind of molecular motions which we call heat vibrations; but there is a vagueness about the terms of such guesses that leaves them clearly within the category of explanations that do not explain.
When it comes to the phenomena of light, we can, as is fitting, see our way a little more clearly, since, thanks to Thomas Young and his successors, we know pretty definitely what light really is. So when we learn that many substances change their color utterly at low temperatures--red things becoming yellow and yellow things white, for example--we can step easily and surely to at least a partial explanation. We know that the color of any object depends simply upon the particular ether waves of the spectrum which that particular substance absorbs; and it does not seem anomalous that molecules packed close together at--180 of temperature should treat the ether waves differently than when relatively wide apart at an ordinary temperature.
Yet, after all, that may not be the clew to the explanation. The packing of the molecules may have nothing to do with it. The real explanation may lie in the change of the ether waves sent out by the vibrating molecule; indeed, the fact that the waves of radiant heat and those of light differ only in amplitude lends color to this latter supposition.
So the explanation of the changed color of the cooled substance is at best a dubious one.
Another interesting light phenomenon is found in the observed fact that very many substances become markedly phosph.o.r.escent at low temperatures.
Thus, according to Professor Dewar, "gelatine, celluloid, paraffine, ivory, horn, and india-rubber become distinctly luminous, with a bluish or greenish phosph.o.r.escence, after cooling to--180 and being stimulated by the electric light." The same thing is true, in varying degrees, of alcohol, nitric acid, glycerine, and of paper, leather, linen, tortoise-sh.e.l.l, and sponge. Pure water is but slightly luminous, whereas impure water glows brightly. On the other hand, alcohol loses its phosph.o.r.escence when a trace of iodine is added to it. In general, colored things are but little phosph.o.r.escent. Thus the white of egg is very brilliant but the yolk much less so. Milk is much brighter than water, and such objects as a white flower, a feather, and egg-sh.e.l.l glow brilliantly. The most remarkable substances of all, says Professor Dewar, whom I am all along quoting, are "the platinocyanides among inorganic compounds and the ketonic compounds among organic. Ammonium platinocyanide, cooled while stimulated by arc light, glows fully at--180; but on warming it glows like a lamp. It seems clear,"
Professor Dewar adds, "that the substance at this low temperature must have acquired increased power of absorption, and it may be that at the same time the factor of molecular friction or damping may have diminished." The cautious terms in which this partial explanation is couched suggest how far we still are from a full understanding of the interesting phenomena of phosph.o.r.escence. That a molecule should be able to vibrate in such a way as to produce the short waves of light, dissevered from the usual linking with the vibrations represented by high temperature, is one of the standing puzzles of physics. And the demonstrated increase of this capacity at very low temperatures only adds to the mystery.
There are at least two of the low-temperature phenomena, however, that seem a little less puzzling--the facts, namely, that cohesion and rigidity of structure are increased when a substance is cooled and that chemical activity is very greatly reduced, in fact almost abolished.
This is quite what one would expect _a priori_--though no wise man would dwell on his expectation in advance of the experiments--since the whole question of liquids and solids _versus_ gases appears to be simply a contest between cohesive forces that are tending to draw the molecules together and the heat vibration which is tending to throw them apart.
As a substance changes from gas to liquid, and from liquid to solid, contracting meantime, simply through the lessening of the heat vibrations of its molecules, we might naturally expect that the solid would become more and more tenacious in structure as its molecules came closer and closer together, and at the same time became less and less active, as happens when the solid is further cooled. And for once experiment justifies the expectation. Professor De-war found that the breaking stress of an iron wire is more than doubled when the wire is cooled to the temperature of liquid air, and all other metals are largely strengthened, though none other to quite the same degree.
He found that a spiral spring of fusible metal, which at ordinary temperature was quickly drawn out into a straight wire by a weight of one ounce, would, when cooled to -182 deg, support a weight of two pounds, and would vibrate like a steel spring so long as it was cool.
A bell of fusible metal has a distinct metallic ring at this low temperature; and b.a.l.l.s of iron, tin, lead, or ivory cooled to -182 deg and dropped from a height, "in all cases have the rebound greatly increased. The flattened surface of the lead is only one-third what it would be at ordinary temperature." "These conditions are due solely to the cooling, and persist only while the low temperature lasts."
If this increased strength and hardness of a contracted metal are what one would expect on molecular principles, the decreased chemical activity at low temperatures is no less natural-seeming, when one reflects how generally chemical phenomena are facilitated by the application of heat. In point of fact, it has been found that at the temperature of liquid hydrogen practically all chemical activity is abolished, the unruly fluorine making the only exception. The explanation hinges on the fact that every atom, of any kind, has power to unite with only a limited number of other atoms. When the "affinities" of an atom are satisfied, no more atoms can enter into the union unless some atoms already there be displaced. Such displacement takes place constantly, under ordinary conditions of temperature, because the vibrating atoms tend to throw themselves apart, and other atoms may spring in to take the places just vacated--such interchange, in fact, const.i.tuting the essence of chemical activity. But when the temperature is reduced the heat-vibration becomes insufficient to throw the atoms apart, hence any unions they chance to have made are permanent, so long as the low temperature is maintained. Thus it is that substances which attack one another eagerly at ordinary temperatures will lie side by side, utterly inert, at the temperature of liquid air.
Under certain conditions, however, most interesting chemical experiments have been made in which the liquefied gases, particularly oxygen, are utilized. Thus Olzewski found that a bit of wood lighted and thrust into liquid oxygen burns as it would in gaseous oxygen, and a red-hot iron wire thrust into the liquid burns and spreads sparks of iron. But more novel still was Dewar's experiment of inserting a small jet of ignited hydrogen into the vessel of liquid oxygen; for the jet continued to burn, forming water, of course, which was carried away as snow. The idea of a gas-jet burning within a liquid, and having snow for smoke, is not the least anomalous of the many strange conceptions that the low-temperature work has made familiar.
PRACTICAL RESULTS AND ANTIc.i.p.aTIONS
Such are some of the strictly scientific results of the low-temperature work. But there are other results of a more directly practical kind--neither more important nor more interesting on that account, to be sure, but more directly appealing to the generality of the non-scientific public. Of these applications, the most patent and the first to be made available was the one forecast by Davy from the very first--namely, the use of liquefied gases in the refrigeration of foods. Long before the more resistant gases had been liquefied, the more manageable ones, such as ammonia and sulphurous acid, had been utilized on a commercial scale for refrigerating purposes. To-day every brewery and every large cold-storage warehouse is supplied with such a refrigerator plant, the temperature being thus regulated as is not otherwise practicable. Many large halls are cooled in a similar manner, and thus made comfortable in the summer. s.h.i.+ps carrying perishables have the safety of their cargoes insured by a refrigerator plant. In all large cities there are ice manufactories using the same method, and of late even relatively small establishments, hotels, and apartment houses have their ice-machine. It seems probable that before long all such buildings and many private dwellings will be provided with a cooling apparatus as regularly as they are now equipped with a heating apparatus.
The exact details of the various refrigerator machines of course vary, but all of them utilize the principles that the laboratory workers first established. Indeed, the entire refrigerator industry, now a.s.suming significant proportions, may be said to be a direct outgrowth of that technical work which Davy and Faraday inaugurated and prosecuted at the Royal Inst.i.tution--a result which would have been most gratifying to the founder of the inst.i.tution could he have forecast it. The usual means of distributing the cooling fluids in the commercial plants is by the familiar iron pipes, not dissimilar in appearance (when not in operation) to the familiar gas, water, and steam pipes. When operating, however, the pipes themselves are soon hidden from view by the thick coating of frost which forms over them. In a moist beer-cellar this coating is often several inches in thickness, giving a very characteristic and unmistakable appearance.
Another commercial use to which refrigerator machines are now put is in the manufacture of various drugs, where absolute purity is desirable.
As different substances congeal at different temperatures, but the same substances at uniform pressure always at the same temperature, a means is afforded of freeing a drug from impurities by freezing, where sometimes the same result cannot be accomplished with like thoroughness by any other practicable means. Indeed, by this means impurities have been detected where not previously suspected. And Professor Ramsay has detected some new elementary substances even, as const.i.tuents of the air, which had previously not been dissociated from the nitrogen with which they are usually mixed.
Such applications of the refrigerator principles as these, however, though of vast commercial importance, are held by many enthusiasts to be but a bagatelle compared with other uses to which liquefied gases may some time be put. Their expectations are based upon the enormous potentialities that are demonstrably stored in even a tiny portion of, say, liquefied air. These are, indeed, truly appalling. Consider, for example, a portion of air at a temperature above its critical point, to which, as in Thilorier's experiments, a pressure of thirty-one tons to the square inch of the encompa.s.sing wall is being applied. Recall that action and reaction are equal, and it is apparent that the gas itself is pus.h.i.+ng back--struggling against being compressed, if you will--with an equal power. Suppose the bulk of the gas is such that at this pressure it occupies a cubical s.p.a.ce six inches on a side--something like the bulk of a child's toy balloon, let us say. Then the total outward pressure which that tiny bulk of gas exerts, in its desperate molecular struggle, is little less than five thousand tons. It would support an enormous building without budging a hair's-breadth. If the building weighed less than five thousand tons it would be lifted by the gas; if much less it would be thrown high into the air as the gas expanded. It gives one a new sense of the power of numbers to feel that infinitesimal atoms, merely by vibrating in unison, could accomplish such a result.
But now suppose our portion of gas, instead of being placed under our hypothetical building, is plunged into a cold medium, which will permit its heat-vibrations to exhaust themselves without being correspondingly restored. Then, presently, the temperature is lowered below the critical point, and, presto! the mad struggle ceases, the atoms lie amicably together, and the gas has become a liquid. What a transformed thing it is now. Instead of pressing out with that enormous force, it has voluntarily contracted as the five thousand tons pressure could not make it do; and it lies there now, limpid and harmless-seeming, in the receptacle, for all the world like so much water.
And, indeed, the comparison with water is more than superficial, for in a cup of water also there are wonderful potentialities, as every steam-engine attests. But an enormous difference, not in principle but in practical applications, exists in the fact that the potentialities of the water cannot be utilized until relatively high temperatures are reached. Costly fuel must be burned and the heat applied to the water before it can avail to do its work. But suppose we were to place our portion of liquid air, limpid and water-like, in the cylinder of a locomotive, where the steam of water ordinarily enters. Then, though no fuel were burned--though the entire engine stood embedded in the snow of an arctic winter--it would be but a few moments before the liquid air would absorb even from this cold medium heat enough to bring it above its critical temperature; and, its atoms now dancing apart once more and re-exerting that enormous pressure, the piston of the engine would be driven back and then the entire cylinder burst into fragments as the gas sought exit. In a word, then, a portion of liquid air has a store of potential energy which can be made kinetic merely by drawing upon the boundless and free supply of heat which is everywhere stored in the atmosphere we breathe and in every substance about us. The difficulty is, not to find fuel with which to vaporize it, as in case of water, but to keep the fuel from finding it whether or no. Were liquid air in sufficient quant.i.ties available, the fuel problem would cease to have any significance. But of course liquid air is not indefinitely available, and exactly here comes the difficulty with the calculations of many enthusiasts who hail liquefied gas as the motive power of the near future. For of course in liquefying the air power has been applied, for the moment wasted, and unless we can get out of the liquid more energy than we have applied to it, there is no economy of power in the transaction. Now the simplest study of the conditions, with the mechanical theory of matter in mind, makes it clear that this is precisely what one can never hope to accomplish. Action and reaction are equal and in opposite directions at all stages of the manipulation, and hence, under the most ideal conditions, we must expect to waste as much work in condensing a gas (in actual practice more) as the condensed substance can do in expanding to the original volume. Those enthusiasts who have thought otherwise, and who have been on the point of perfecting an apparatus which will readily and cheaply produce liquid air after the first portion is produced, are really but following the old perpetual-motion-machine will-o'-the-wisp.
It does not at all follow from this, however, that the energies of liquefied air may not be utilized with enormous advantage. It is not always the cheapest form of power-transformer that is the best for all purposes, as the use of the electrical storage battery shows. And so it is quite within the possibilities that a mult.i.tude of uses may be found for the employment of liquid air as a motive power, in which its condensed form, its transportability or other properties will give it precedence over steam or electricity. It has been suggested, for example, that liquefied gas would seem to afford the motive power par excellence for the flying-machine, once that elusive vehicle is well in harness, since one of the greatest problems here is to reduce the weight of the motor apparatus. In a less degree the same problem enters into the calculations of s.h.i.+ps, particularly s.h.i.+ps of war; and with them also it may come to pa.s.s that a store of liquid air (or other gas) may come to take the place of a far heavier store of coal. It is even within the possibilities that the explosive powers of the same liquid may take the place of the great magazines of powder now carried on war-s.h.i.+ps; for, under certain conditions, the liquefied gas will expand with explosive suddenness and violence, an "explosion" being in any case only a very sudden expansion of a confined gas. The use of the compressed air in the dynamite guns, as demonstrated in the Cuban campaign, is a step in this direction. And, indeed, the use of compressed air in many commercial fields already competing with steam and electricity is a step towards the use of air still further compressed, and cooled, meantime, to a condition of liquidity. The enormous advantages of the air actually liquefied, and so for the moment quiescent, over the air merely compressed, and hence requiring a powerful retort to hold it, are patent at a glance. But, on the other hand, the difficulty of keeping it liquid is a disadvantage that is equally patent. How the balance will be struck between these contending advantages and disadvantages it remains for the practical engineering inventors of the future--the near future, probably--to demonstrate.
Meantime there is another line of application of the ideas which the low-temperature work has brought into prominence which has a peculiar interest in the present connection because of its singularly Rumfordian cast, so to speak, I mean the idea of the insulation of cooled or heated objects in the ordinary affairs of life, as, for example, in cooking.
The subject was a veritable hobby with the founder of the Royal Inst.i.tution all his life. He studied the heat-transmitting and heat-reflecting properties of various substances, including such directly practical applications as rough surfaces _versus_ smooth surfaces for stoves, the best color for clothing in summer and in winter, and the like. He promulgated his ideas far and wide, and demonstrated all over Europe the extreme wastefulness of current methods of using fuel. To a certain extent his ideas were adopted everywhere, yet on the whole the public proved singularly apathetic; and, especially in America, an astounding wastefulness in the use of fuel is the general custom now as it was a century ago. A French cook will prepare an entire dinner with a splinter of wood, a handful of charcoal, and a half-shovelful of c.o.ke, while the same fuel would barely suffice to kindle the fire in an American cook-stove. Even more wonderful is the German stove, with its great bulk of brick and mortar and its glazed tile surface, in which, by keeping the heat in the room instead of sending it up the chimney, a few bits of compressed coal do the work of a hodful.
It is one merit of the low-temperature work, I repeat, to have called attention to the possibilities of heat insulation in application to "the useful purposes of life." If Professor Dewar's vacuum vessel can reduce the heat-transmitting capacity of a vessel by almost ninety-seven per cent., why should not the same principle, in modified form, be applied to various household appliances--to ice-boxes, for example, and to cooking utensils, even to ovens and cook-stoves? Even in the construction of the walls of houses the principles of heat insulation might advantageously be given far more attention than is usual at present; and no doubt will be so soon as the European sense of economy shall be brought home to the people of the land of progress and inventions. The principles to be applied are already clearly to hand, thanks largely to the technical workers with low temperatures. It remains now for the practical inventors to make the "application to the useful purposes of life." The technical scientists, ignoring the example which Rumford and a few others have set, have usually no concern with such uninteresting concerns.
For the technical scientists themselves, however, the low-temperature field is still full of inviting possibilities of a strictly technical kind. The last gas has indeed been liquefied, but that by no means implies the last stage of discovery. With the successive conquest of this gas and of that, lower and lower levels of temperature have been reached, but the final goal still lies well beyond. This is the north pole of the physicist's world, the absolute zero of temperature--the point at which the heat-vibrations of matter are supposed to be absolutely stilled. Theoretically this point lies 2720 below the Centigrade zero. With the liquefaction of hydrogen, a temperature of about -253 deg or -254 deg Centigrade has been reached. So the gap seems not so very great. But like the gap that separated Nansen from the geographical pole, it is a very hard road to travel. How to compa.s.s it will be the study of all the low-temperature explorers in the immediate future. Who will first reach it, and when, and how, are questions for the future to decide.
And when the goal is reached, what will be revealed? That is a question as full of fascination for the physicist as the north-pole mystery has ever been for the generality of mankind. In the one case as in the other, any attempt to answer it to-day must partake largely of the nature of a guess, yet certain forecasts may be made with reasonable probability. Thus it can hardly be doubted that at the absolute zero all matter will have the form which we term solid; and, moreover, a degree of solidity, of tenacity and compactness greater than ever otherwise attained. All chemical activity will presumably have ceased, and any existing compound will retain unaltered its chemical composition so long as absolute zero pertains; though in many, if not in all cases, the tangible properties of the substance--its color, for example, and perhaps its crystalline texture--will be so altered as to be no longer recognizable by ordinary standards, any more than one would ordinarily recognize a ma.s.s of snowlike crystals as air.
A History of Science Volume V Part 2
You're reading novel A History of Science Volume V Part 2 online at LightNovelFree.com. You can use the follow function to bookmark your favorite novel ( Only for registered users ). If you find any errors ( broken links, can't load photos, etc.. ), Please let us know so we can fix it as soon as possible. And when you start a conversation or debate about a certain topic with other people, please do not offend them just because you don't like their opinions.
A History of Science Volume V Part 2 summary
You're reading A History of Science Volume V Part 2. This novel has been translated by Updating. Author: Henry Smith Williams already has 736 views.
It's great if you read and follow any novel on our website. We promise you that we'll bring you the latest, hottest novel everyday and FREE.
LightNovelFree.com is a most smartest website for reading novel online, it can automatic resize images to fit your pc screen, even on your mobile. Experience now by using your smartphone and access to LightNovelFree.com
RECENTLY UPDATED NOVEL

My Girlfriend is a Zombie
My Girlfriend is a Zombie Chapter 844: Xiao Bai in the Grass View : 2,302,128
Alchemy Emperor Of The Divine Dao
Alchemy Emperor Of The Divine Dao Chapter 4357 Another Deal View : 13,711,374
Cultivating In Secret Beside A Demoness
Cultivating In Secret Beside A Demoness Chapter 1318: Senior, I'm Tired of Standing (1) View : 510,550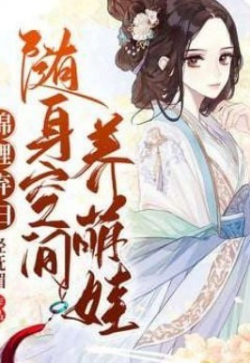
Raising My Children With My Personal Spatial Ability
Raising My Children With My Personal Spatial Ability Chapter 1805 I Actually Don't Want to... View : 1,248,991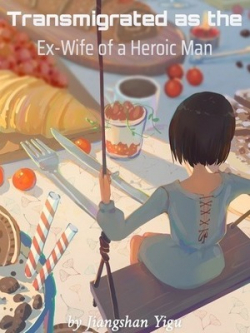
Transmigrated as the Ex-Wife of a Heroic Man
Transmigrated as the Ex-Wife of a Heroic Man Chapter 1313: Refreshing One's Worldview View : 578,989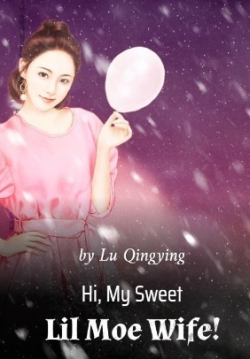