The Hidden Reality Part 10
You’re reading novel The Hidden Reality Part 10 online at LightNovelFree.com. Please use the follow button to get notification about the latest chapter next time when you visit LightNovelFree.com. Use F11 button to read novel in full-screen(PC only). Drop by anytime you want to read free – fast – latest novel. It’s great if you could leave a comment, share your opinion about the new chapters, new novel with others on the internet. We’ll do our best to bring you the finest, latest novel everyday. Enjoy!
Maldacena's first step was to confine his mathematical attention to strings that have low energy-that is, ones that vibrate relatively slowly. Here's why: the force of gravity between any two objects is proportional to the ma.s.s of each; the same is true for the force of gravity acting between any two strings. Strings that have low energy have small ma.s.s, and so they hardly respond to gravity at all. By focusing on low energy strings, Maldacena was thus suppressing gravity's influence. That yielded a substantial simplification. In string theory, as we've seen (Chapter 5), gravity is transmitted from place to place by closed loops. Suppressing the force of gravity was therefore tantamount to suppressing the influence of closed strings on anything they might encounter-most notably, the open string snippets living on the brane stack. By ensuring that the two kinds of strings, open snippets and closed loops, wouldn't affect each other, Maldacena was ensuring that they could be a.n.a.lyzed independently.
Figure 9.4 A collection of closely s.p.a.ced three-branes with open strings confined to the brane surfaces, and closed strings moving through the "bulk." A collection of closely s.p.a.ced three-branes with open strings confined to the brane surfaces, and closed strings moving through the "bulk."
Maldacena then changed gears and suggested thinking about the very same situation from a different perspective. Rather than treat the three-branes as a substrate that supports the motion of open strings, he encouraged viewing them as a single object, which has its own intrinsic ma.s.s and hence warps s.p.a.ce and time in its vicinity. Maldacena was fortunate that previous research, by a number of physicists, had laid the groundwork for this alternative perspective. The earlier works had established that as you stack more and more branes together, their collective gravitational field grows ever stronger. Ultimately, the slab of branes behaves much like a black hole, but one that's brane-shaped, and so is called a black brane black brane. As with a more ordinary black hole, if you get too close to a black brane, you can't escape. And, as is also the case with an ordinary black hole, if you stay far away but are watching something approach a black brane, the light you'll receive will be exhausted from its having fought against the black brane's gravity. This will make the object appear to have ever less energy and to be moving ever slower.14 From this second perspective, Maldacena again focused on the low-energy features of a universe containing such a black slab. Much as he had when working on the first perspective, he realized that the low-energy physics involved two components that could be a.n.a.lyzed independently. Slowly vibrating closed strings, moving anywhere in the bulk of s.p.a.ce, are the most obvious low-energy carriers. The second component relies on the presence of the black brane. Imagine you are far from the black brane and have in your possession a closed string that's vibrating with an arbitrarily large amount of energy. Then, imagine lowering the string toward the event horizon while you maintain a safe distance. As recalled above, the black brane will make the string's energy appear ever lower; the light you'll receive will make the string look as though it's in a slow-motion movie. The second low-energy carriers are thus any and all vibrating strings that are sufficiently close to the black brane's event horizon.
Maldacena's final move was to compare the two perspectives. He noted that because they describe the same brane stack, only from different points of view, they must agree. Each description involves low-energy closed strings moving through the bulk of s.p.a.ce, so this part of the agreement is manifest. But the remaining part of each description must also agree.
And that proves astonis.h.i.+ng.
The remaining part of the first description consists of low-energy open strings moving on the three-branes. We recall from Chapter 4 Chapter 4 that low-energy strings are well described by point particle quantum field theory, and that is the case here. The particular kind of quantum field theory involves a number of sophisticated mathematical ingredients (and it has an ungainly characterization: that low-energy strings are well described by point particle quantum field theory, and that is the case here. The particular kind of quantum field theory involves a number of sophisticated mathematical ingredients (and it has an ungainly characterization: conformally invariant supersymmetric quantum gauge field theory conformally invariant supersymmetric quantum gauge field theory), but two vital characteristics are readily understood. The absence of closed strings ensures the absence of the gravitational field. And, because the strings can move only on the tightly sandwiched three-dimensional branes, the quantum field theory lives in three spatial dimensions (in addition to the one dimension of time, for a total of four s.p.a.cetime dimensions).
The remaining part of the second description consists of closed strings, executing any vibrational pattern, as long as they are close enough to the black branes' event horizon to appear lethargic-that is, to appear to have low energy. Such strings, although limited in how far they stray from the black stack, still vibrate and move through nine dimensions of s.p.a.ce (in addition to one dimension of time, for a total of ten s.p.a.cetime dimensions). And because this sector is built from closed strings, it contains the force of gravity.
However different the two perspectives might seem, they're describing one and the same physical situation, so they must agree. This leads to a thoroughly bizarre conclusion. A particular nongravitational, point particle nongravitational, point particle quantum field theory in quantum field theory in four four s.p.a.cetime dimensions (the first perspective) describes the same physics as s.p.a.cetime dimensions (the first perspective) describes the same physics as strings, including gravity strings, including gravity, moving through a particular swath of ten ten s.p.a.cetime dimensions (the second perspective). This would seem as far-fetched as claiming...Well, honestly, I've tried, and I can't come up with any two things in the real world more dissimilar than these two theories. But Maldacena followed the math, in the manner we've outlined, and ran smack into this conclusion. s.p.a.cetime dimensions (the second perspective). This would seem as far-fetched as claiming...Well, honestly, I've tried, and I can't come up with any two things in the real world more dissimilar than these two theories. But Maldacena followed the math, in the manner we've outlined, and ran smack into this conclusion.
The sheer strangeness of the result-and the audacity of the claim-isn't lessened by the fact that it takes but a moment to place it within the line of thought developed earlier in this chapter. As schematically ill.u.s.trated in Figure 9.5 Figure 9.5, the gravity of the black brane slab imparts a curved shape to the ten-dimensional s.p.a.cetime swath in its vicinity (the details are secondary, but the curved s.p.a.cetime is called antiDe Sitter five-s.p.a.ce times the five sphere antiDe Sitter five-s.p.a.ce times the five sphere); the black brane slab is itself the boundary of this s.p.a.ce. And so, Maldacena's result is that string theory within the bulk bulk of this s.p.a.cetime shape is identical to a quantum field theory living on its of this s.p.a.cetime shape is identical to a quantum field theory living on its boundary. boundary.15 This is holography come to life.
Maldacena had built a self-contained mathematical laboratory in which, among other things, physicists could explore in concrete detail a holographic realization of physical law. Within a few months, two papers, one by Edward Witten and one by Steven Gubser, Igor Klebanov, and Alexander Polyakov, supplied the next level of understanding. They established a precise mathematical dictionary for translating between the two perspectives: given a physical process on the brane boundary, the dictionary showed how it would appear in the bulk interior, and vice versa. In a hypothetical universe, then, the dictionary rendered the holographic principle explicit. On the boundary of this universe, information is embodied by quantum fields. When the information is translated by the mathematical dictionary, it reads as a story of stringy phenomena happening in the universe's interior.
Figure 9.5 A schematic ill.u.s.tration of the duality between string theory operating in the interior of a particular s.p.a.cetime and quantum field theory operating on the boundary of that s.p.a.cetime A schematic ill.u.s.tration of the duality between string theory operating in the interior of a particular s.p.a.cetime and quantum field theory operating on the boundary of that s.p.a.cetime.
Figure 9.6 The holographic equivalence applied to a black hole in the bulk of s.p.a.cetime yields a hot bath of particles and radiation on the region's boundary The holographic equivalence applied to a black hole in the bulk of s.p.a.cetime yields a hot bath of particles and radiation on the region's boundary.
The dictionary itself renders the holographic metaphor all the more appropriate. An everyday hologram bears no resemblance to the three-dimensional image it produces. On its surface appear only various lines, arcs, and swirls etched into the plastic. Yet a complex transformation, carried out operationally by s.h.i.+ning a laser through the plastic, turns those markings into a recognizable three-dimensional image. Which means that the plastic hologram and the three-dimensional image embody the same data, even though the information in one is unrecognizable from the perspective of the other. Similarly, examination of the quantum field theory on the boundary of Maldacena's universe shows that it bears no obvious resemblance to the string theory inhabiting the interior. If a physicist were presented with both theories, not being told of the connections we've now laid out, he or she would more than likely conclude that they were unrelated. Nevertheless, the mathematical dictionary linking the two-functioning as a laser does for ordinary holograms-makes explicit that anything taking place in one has an incarnation in the other. At the same time, examination of the dictionary reveals that just as with a real hologram, the information in each appears scrambled on translation into the other's language.
As a particularly impressive example, Witten investigated what an ordinary black hole in the interior of Maldacena's universe would look like from the perspective of the boundary theory. Remember, the boundary theory does not include gravity, and so a black hole necessarily translates into something very unlike a black hole. Witten's result showed that much as the Wizard of Oz's frightening visage was produced by an ordinary man, a rapacious black hole is the holographic projection of something equally ordinary: a bath of hot particles in the boundary theory (Figure 9.6). Like a real hologram and the image it generates, the two theories-a black hole in the interior and a hot quantum field theory on the boundary-bear no apparent resemblance to each other, and yet they embody identical information.*
In Plato's parable of the cave, our senses are privy only to a flattened, diminished version of the true, more richly textured, reality. Maldacena's flattened world is very different. Far from being diminished, it tells the full story. It's a profoundly different story from the one we're used to. But his flattened world may well be the primary narrator.
Parallel Universes or Parallel Mathematics?
Maldacena's result, and the many others it has sp.a.w.ned in the years since, is deemed conjectural. Because the mathematics is tremendously difficult, fas.h.i.+oning an airtight argument remains elusive. But the holographic ideas have been subject to a great many stringent mathematical tests; having come through unscathed, they've been propelled into mainstream thought among physicists searching for the deep roots of natural laws.
One factor contributing to the difficulty of rigorously proving that the boundary and bulk worlds are disguised versions of one another highlights why the result, if true, is so powerful. I described in Chapter 5 Chapter 5 how physicists more often than not rely on approximation techniques, the perturbative methods that I outlined (recall the lottery example with Ralph and Alice). I also emphasized that such methods are accurate only if the relevant coupling constant is a small number. In a.n.a.lyzing the relations.h.i.+p between quantum field theory on the boundary and string theory in the bulk, Maldacena realized that when the coupling of one theory was small, that of the other was large, and vice versa. The natural test, and a possible means of proving that the two theories are secretly identical, is to perform independent calculations in each theory and then check for equality. But this is difficult to do, since when perturbative methods work for one, they fail for the other. how physicists more often than not rely on approximation techniques, the perturbative methods that I outlined (recall the lottery example with Ralph and Alice). I also emphasized that such methods are accurate only if the relevant coupling constant is a small number. In a.n.a.lyzing the relations.h.i.+p between quantum field theory on the boundary and string theory in the bulk, Maldacena realized that when the coupling of one theory was small, that of the other was large, and vice versa. The natural test, and a possible means of proving that the two theories are secretly identical, is to perform independent calculations in each theory and then check for equality. But this is difficult to do, since when perturbative methods work for one, they fail for the other.16 However, if you accept Maldacena's more abstract argument, as outlined in the previous section, the perturbative vice becomes a calculational virtue. Much as we found with the string dualities in Chapter 5 Chapter 5, the bulk-boundary dictionary translates daunting calculations, beset by a large coupling, in one framework into straightforward calculations, with a small coupling in the other. In recent years, this has been parlayed into results that may be experimentally testable.
At the Relativistic Heavy Ion Collider (RHIC) in Brookhaven, New York, gold nuclei are slammed into each other at just shy of light speed. Because the nuclei contain many protons and neutrons, the collisions create a commotion of particles that can be more than 200,000 times as hot as the sun's core. That's hot enough to melt the protons and neutrons into a fluid of quarks and the gluons that act between them. Physicists have exerted great effort to understand this fluidlike phase, called the quark gluon plasma quark gluon plasma, because it's likely that matter briefly a.s.sumed this form soon after the big bang.
The challenge is that the quantum field theory (quantum chromodynamics) describing the hot soup of quarks and gluons has a large value for its coupling constant, and that compromises the accuracy of perturbative methods. Ingenious techniques have been developed to skirt this hurdle, but experimental measurements continue to controvert some of the theoretical results. For example, as any fluid flows-be it water, mola.s.ses, or the quark gluon plasma-each layer of the fluid exerts a drag force on the layers flowing above and below. The drag force is known as shear viscosity shear viscosity. Experiments at RHIC measured the shear viscosity of the quark gluon plasma, and the results are far smaller than those predicted by the perturbative quantum field theory calculations.
Here's a possible way forward. In introducing the holographic principle, the perspective I've taken is to imagine that everything we experience lies in the interior of s.p.a.cetime, with the unexpected twist being processes, mirroring those experiences, which take place on a distant boundary. Let's reverse that perspective. Imagine that our universe-or, more precisely, the quarks and gluons in our universe-lives on the boundary, and so that's where the RHIC experiments take place. Now invoke Maldacena. His result shows that the RHIC experiments (described by quantum field theory) have an alternative mathematical description in terms of strings moving in the bulk. The details are involved but the power of the rephrasing is immediate: difficult calculations in the boundary description (where the coupling is large) are translated into easier calculations in the bulk description (where the coupling is small).17 Pavel Kovtun, Andrei Starinets, and Dam Son did the math, and the results they found come impressively close to the experimental data. This pioneering work has motivated an army of theoreticians to undertake many other string theory calculations in an effort to make contact with RHIC observations, driving forward a vigorous interplay between theory and experiment-a welcome novelty for string theorists.
Bear in mind that the boundary theory doesn't model our universe fully since, for example, it doesn't contain the gravitational force. This doesn't compromise contact with RHIC data because in those experiments the particles have such small ma.s.s (even when traveling near light speed) that the gravitational force plays virtually no role. But it does make clear that in this application string theory is not being used as a "theory of everything"; instead, string theory provides a new calculational tool for breaking through obstacles that have impeded more traditional methods. Conservatively, a.n.a.lyzing quarks and gluons by using a higher dimensional theory of strings can be viewed as a potent string-based mathematical trick. Less conservatively, one can imagine that the higher dimensional string description is, in some yet to be understood way, physically real.
Regardless of perspective, conservative or not, the resulting confluence of mathematical results with experimental observations is extremely impressive. I am not a fan of hyperbole, but I view these developments as among the most exciting advances in decades. Mathematical manipulations that utilize strings moving through a particular ten-dimensional s.p.a.cetime tell us something about quarks and gluons living in a four-dimensional s.p.a.cetime-and the "something" the calculations tell us seems to be borne out by experiments.
Coda: The Future of String Theory.
The developments we've covered in this chapter transcend evaluations of string theory. From Wheeler's emphasis on a.n.a.lyzing the universe in terms of information, to the recognition that entropy is a measure of hidden information, to the reconciliation between the Second Law of Thermodynamics and black holes, to the realization that black holes store entropy on their surface, to the understanding that black holes set a maximum for the amount of information that can occupy a given region of s.p.a.ce, we've followed a winding road across many decades and traversed an intricate web of results. The journey has been full of remarkable insights, and has led us to a new unifying idea-the holographic principle. The principle, as we've seen, suggests that the phenomena we witness are mirrored on a thin, distant bounding surface. Looking to the future, I suspect that the holographic principle will be a beacon for physicists well into the twenty-first century.
That string theory embraces the holographic principle, and provides concrete examples of holographic parallel worlds, is a testament to how cutting-edge developments are coming together in a powerful synthesis. That these examples have provided the basis for explicit calculations, some of whose results can be compared with results from real-world experiments, is a gratifying step toward making contact with observable reality. But within string theory itself, there's a broader frame within which these developments should be seen.
For nearly thirty years after the initial discovery of string theory, physicists lacked a full mathematical definition of the theory. Early string theorists laid out the essential ideas of vibrating strings and extra dimensions, but even after decades of further work, the mathematical foundations of the theory remained approximate and thus incomplete. Maldacena's insight represents major progress. The species of quantum field theory Maldacena identified as living on the boundary is among the mathematically best understood of those particle physicists have studied since the middle of the twentieth century. It does not include gravity, and that's a big plus since, as we've seen, trying to bring general relativity directly into quantum field theory is like setting a campfire in a gunpowder factory. We've now learned that this mathematically friendly, nongravitational quantum field theory generates generates string theory-a theory that contains gravity-holographically. Operating way out on the boundary of a universe with the specific shape schematically ill.u.s.trated in string theory-a theory that contains gravity-holographically. Operating way out on the boundary of a universe with the specific shape schematically ill.u.s.trated in Figure 9.5 Figure 9.5, this quantum field theory embodies all physical features, processes, and interactions of strings that move within the interior, a link made explicit through the dictionary translating phenomena between the two. And since we have a sure-footed mathematical definition of the boundary quantum field theory, we can use it as a mathematical definition of string theory we can use it as a mathematical definition of string theory, at least for strings moving within this s.p.a.cetime shape. The holographic parallel universes may thus be more than a potential outgrowth of fundamental laws; they may be part of the very definition of the fundamental laws.18 When I introduced string theory in Chapter 4 Chapter 4, I noted that it fit the venerable pattern of providing a new approach to nature's laws that, nevertheless, did not erase past theories. The results we've now described take this observation to a whole different level. String theory doesn't just reduce to quantum field theory in certain circ.u.mstances. Maldacena's result suggests that string theory and quantum field theory are equivalent approaches expressed in different languages. The translation between them is complicated, which is why it took more than forty years for this connection to come to light. But if Maldacena's insights are fully valid, as all available evidence attests, string theory and quantum field theory may very well be two sides of the same coin.
Physicists are working hard to generalize the methods so they might apply to a universe with any shape; if string theory is right, that would include ours. But even with the current limitations, finally having a firm formulation of a theory we've worked on for many years is an essential foundation for future progress. It is surely enough to make many a physicist sing and dance.
*This loose definition will suffice for now; in a moment, I'll be more precise.
*In Chapter 3 Chapter 3, we discussed how the energy embodied by a gravitational field can be negative; this energy, however, is potential energy. The energy we're discussing here, kinetic energy, comes from the electron's ma.s.s and its motion. In cla.s.sical physics this has to be positive.
*Besides flipping the coins, you could also swap around their locations, but for the purpose of ill.u.s.trating the main ideas, we can safely ignore this complication.
*If you're interested in the full story, I highly recommend Leonard Susskind's excellent book The Black Hole Wars The Black Hole Wars.
*The reader familiar with black holes will note that even without the quantum considerations that lead to Hawking radiation, the two perspectives would differ with regards to the rate of time's pa.s.sage. Hawking radiation makes the perspectives yet more distinct.
*There is a related story that I've not told in this chapter, to do with a long-standing debate regarding whether black holes require a modification of quantum mechanics-whether, by swallowing information, they upend the ability to fully evolve probability waves forward in time. A one-sentence summary is that Witten's result, by establis.h.i.+ng an equivalence between a black hole and a physical situation that does not not destroy information (a hot quantum field theory), supplied conclusive evidence that all information that falls into a black hole is ultimately available to the outside world. Quantum mechanics needs no modification. This application of Maldacena's discovery also establishes that the boundary theory provides a full description of the information (entropy) stored on a black hole's surface. destroy information (a hot quantum field theory), supplied conclusive evidence that all information that falls into a black hole is ultimately available to the outside world. Quantum mechanics needs no modification. This application of Maldacena's discovery also establishes that the boundary theory provides a full description of the information (entropy) stored on a black hole's surface.
CHAPTER 10.
Universes, Computers, and Mathematical Reality.
The Simulated and the Ultimate Multiverses.
The parallel universe theories we considered in previous chapters emerged from mathematical laws developed by physicists in their pursuit of nature's deepest workings. The credence accorded one set of laws or another varies widely-quantum mechanics is viewed as established fact; inflationary cosmology has observational support; string theory is thoroughly speculative-as does the type and logical necessity of the parallel worlds a.s.sociated with each. But the pattern is clear. When we hand over the steering wheel to the mathematical underpinnings of the major proposed physical laws, we're driven time and again to some version of parallel worlds.
Let's now change tack. What happens if we seize the wheel? Can we humans manipulate the cosmic unfolding to volitionally create universes parallel to our own? If you believe, as I do, that the behavior of living beings is dictated by nature's laws, then you may see this as no change of tack at all but simply as a narrowing of perspective, to the impact of physical law when funneled through human activity. This line of thought quickly engages th.o.r.n.y issues such as the age-old debate about determinism and free will, but that's not a direction in which I want to head. Rather, my question is this: With the same sense of intent and control you feel when you choose a movie or a meal, might you create a universe?
The question sounds outlandish. And it is. I'll tip you off now that in addressing it we will find ourselves in territory even more speculative than what we've already covered, and considering where we've been, that says a lot. But let's have a little fun and see where it takes us. Let me lay out the perspective I'll take. In contemplating universe creation, I'm less interested in practical constraints than in the possibilities made available by the laws of physics. So, when I speak of "you" creating a universe, what I really mean is you, or a distant descendant, or an army of such descendants possibly millennia down the road. These present or future humans will still be subject to the laws of physics, but I will imagine that they're in possession of arbitrarily advanced technologies. I will also consider the creation of two distinct types of universes. The first type comprises the usual universes, ones that encompa.s.s an expanse of s.p.a.ce and are filled with various forms of matter and energy. The second kind is less tangible: virtual computer-generated universes. The discussion will also naturally forge a link to a third multiverse proposal. This variety does not originate from thinking about universe creation, per se, but instead addresses the question of whether mathematics is "real" or is instead created by the mind.
To Create a Universe.
Despite uncertainties in delineating the composition of the universe-What is the dark energy? What is the full list of fundamental particulate ingredients?-scientists are confident that were you to weigh everything that's within our cosmic horizon, the tally would come in at about 10 billion billion billion billion billion billion grams. If the contents weighed significantly more or less than this, their gravitational influence on the cosmic microwave background radiation would cause the splotches in Figure 3.4 Figure 3.4 to be much larger or smaller, and that would conflict with refined measurements of their angular size. But the precise weight of the observable universe is secondary; my point is that it's huge. So huge that the notion of us humans creating another such realm seems utterly fatuous. to be much larger or smaller, and that would conflict with refined measurements of their angular size. But the precise weight of the observable universe is secondary; my point is that it's huge. So huge that the notion of us humans creating another such realm seems utterly fatuous.
Using big bang cosmology as our blueprint for universe formation, we find no guidance on how to clear this hurdle. In the standard big bang theory, the observable universe was ever-smaller at ever-earlier times, but the stupendous quant.i.ties of matter and energy we now measure were always present; they were just squeezed into an ever-smaller volume. If you want a universe like the one we see today, you have to start with raw material whose ma.s.s and energy are those we see today. The big bang theory takes such raw material as an unexplained given.1 In broad strokes, then, the big bang's instructions for creating a universe like ours require that we gather a gargantuan amount of ma.s.s and compress it to a fantastically small size. But having achieved that, however improbable, we would face another challenge. How do we ignite the bang? It's an obstacle that becomes only more daunting when we recall that the big bang is not an explosion that takes place within a static region of s.p.a.ce; the big bang propels the expansion of s.p.a.ce itself.
If the big bang theory were the pinnacle of cosmological thought, the scientific pursuit of universe creation would stop here. But it's not. We've seen that the big bang theory has given way to the more robust inflationary cosmology, and inflation offers a strategy for going forward. With a powerful outward burst of spatial expansion being its trademark, the inflationary theory puts a bang in the big bang, and a big one at that; according to inflation, an anti-gravity blast is what set the outward expansion of s.p.a.ce in motion. Of equal importance, as we'll now see, inflation establishes that vast amounts of matter can be created created from the most modest of seeds. from the most modest of seeds.
Recall from Chapter 3 Chapter 3 that in the inflationary approach, a universe like ours-a hole in the cosmic Swiss cheese-formed when the inflaton's value rolled down its potential energy curve, bringing to a close the phenomenal outward surge in our vicinity. As the inflaton's value dropped, the energy it contained was transformed into a bath of particles uniformly filling our bubble. That's where the matter we see originated. Progress, for sure, but the insight raises the next question: What's the source of the inflaton's energy? that in the inflationary approach, a universe like ours-a hole in the cosmic Swiss cheese-formed when the inflaton's value rolled down its potential energy curve, bringing to a close the phenomenal outward surge in our vicinity. As the inflaton's value dropped, the energy it contained was transformed into a bath of particles uniformly filling our bubble. That's where the matter we see originated. Progress, for sure, but the insight raises the next question: What's the source of the inflaton's energy?
It comes from gravity. Remember that inflationary expansion is much like viral replication: a high-valued inflaton field drives the region it inhabits to rapidly grow, and in doing so creates an increasingly large spatial volume that is itself infused with a high-valued inflaton field. And because a uniform inflaton field contributes a constant energy per unit volume, the larger the volume it fills, the more energy it embodies. The driving force behind the expansion is gravity-in its repulsive guise-and so gravity is the source of the ever-larger energy the region contains.
Inflationary cosmology can thus be thought of as creating a sustained energy flow from the gravitational field to the inflaton field. This might seem like one more pa.s.sing of the energy buck-where does gravity get its its energy?-but the situation is a good deal better than that. Gravity is different from the other forces because where there's gravity, there's a virtually unlimited reservoir of energy. It's a familiar idea expressed in unfamiliar language. When you jump off a cliff, your kinetic energy-the energy of your motion-gets ever larger. Gravity, the force driving your motion, is the energy's source. In any realistic situation, you will hit the ground, but in principle you could fall arbitrarily far, tumbling down an increasingly long rabbit hole, while your kinetic energy grows ever larger. The reason gravity can supply such unlimited quant.i.ties of energy is that, much like the U.S. Treasury, it has no fear of debt. As you fall and your energy gets ever more positive, gravity compensates by its energy becoming ever more negative. You know intuitively that the gravitational energy is negative because to climb out of the rabbit hole, you need to exert positive energy-pus.h.i.+ng with your legs, pulling with your arms; that's how you repay the energy debt gravity incurred on your behalf. energy?-but the situation is a good deal better than that. Gravity is different from the other forces because where there's gravity, there's a virtually unlimited reservoir of energy. It's a familiar idea expressed in unfamiliar language. When you jump off a cliff, your kinetic energy-the energy of your motion-gets ever larger. Gravity, the force driving your motion, is the energy's source. In any realistic situation, you will hit the ground, but in principle you could fall arbitrarily far, tumbling down an increasingly long rabbit hole, while your kinetic energy grows ever larger. The reason gravity can supply such unlimited quant.i.ties of energy is that, much like the U.S. Treasury, it has no fear of debt. As you fall and your energy gets ever more positive, gravity compensates by its energy becoming ever more negative. You know intuitively that the gravitational energy is negative because to climb out of the rabbit hole, you need to exert positive energy-pus.h.i.+ng with your legs, pulling with your arms; that's how you repay the energy debt gravity incurred on your behalf.2 The essential conclusion is that as an inflaton-filled region rapidly grows, the inflaton extracts energy from the gravitational field's inexhaustible resources, resulting in the region's energy rapidly growing too. And because the inflaton field supplies the energy that's converted into ordinary matter, inflationary cosmology-unlike the big bang model-does not need to posit the raw material for generating planets, stars, and galaxies. Gravity is matter's sugar daddy.
The only independent energy budget required by inflationary cosmology is what's needed to create an initial inflationary seed, a small spherical nugget of s.p.a.ce filled with a high-valued inflaton field that gets the inflationary expansion rolling in the first place. When you put in numbers, the equations show that the nugget need be only about 1026 centimeters across and filled with an inflaton field whose energy, when converted to ma.s.s, would weigh less than ten grams. centimeters across and filled with an inflaton field whose energy, when converted to ma.s.s, would weigh less than ten grams.3 Such a tiny seed would, faster than a flash, undergo spectacular expansion, growing far larger than the observable universe while harboring ever-increasing energy. The inflaton's total energy would quickly soar beyond what's necessary to generate all the stars in all the galaxies we observe. And so, with inflation in the cosmological driver's seat, the impossible starting point of the big bang's recipe-gather more than 10 Such a tiny seed would, faster than a flash, undergo spectacular expansion, growing far larger than the observable universe while harboring ever-increasing energy. The inflaton's total energy would quickly soar beyond what's necessary to generate all the stars in all the galaxies we observe. And so, with inflation in the cosmological driver's seat, the impossible starting point of the big bang's recipe-gather more than 1055 grams and squeeze the whole lot into an infinitesimally small speck-is radically transformed. Gather ten grams of inflaton field and squeeze it into a lump that's about 10 grams and squeeze the whole lot into an infinitesimally small speck-is radically transformed. Gather ten grams of inflaton field and squeeze it into a lump that's about 1026 centimeters across. That's a lump you could put in your wallet. centimeters across. That's a lump you could put in your wallet.
This approach, nevertheless, presents daunting challenges. For one thing, the inflaton remains a purely hypothetical field. Cosmologists freely incorporate the inflaton field into their equations, but unlike with electron and quark fields, there is as yet no evidence that the inflaton field exists. For another, even if the inflaton proves real, and even if we one day develop the means to manipulate it much as we do the electromagnetic field, still the density density of the requisite inflaton seed would be enormous: about 10 of the requisite inflaton seed would be enormous: about 1067 times that of an atomic nucleus. Although the seed would weigh less than a handful of popcorn, the compressive force we would need to apply is trillions and trillions of times beyond what we can now muster. times that of an atomic nucleus. Although the seed would weigh less than a handful of popcorn, the compressive force we would need to apply is trillions and trillions of times beyond what we can now muster.
But this is just the kind of technological hurdle that we're imagining an arbitrarily advanced civilization might one day overcome. So, if our distant descendants one day harness the inflaton field and develop extraordinary compressors capable of producing such dense nuggets, will we have attained the status of universe creators? And, as we contemplate such a step toward Olympus, should we worry that if we artificially set off new inflationary realms, our own corner of s.p.a.ce may be swallowed by the ballooning expanse? Alan Guth and a number of collaborators investigated these questions in a series of papers, and found both good news and bad. Start with the last question, as that's where we'll find the good news.
Guth, together with Steven Blau and Eduardo Guendelman, showed that there's no need to be concerned about an artificial phase of inflationary expansion ripping through our existing environment. The reason has to do with pressure. If an inflationary seed were created in the laboratory, it would harbor the inflaton field's characteristic positive energy and negative pressure, but it would be surrounded by ordinary s.p.a.ce in which the inflaton field's value, and its pressure, would be zero (or nearly so).
We usually don't ascribe much power to zero, but in this case zero makes all the difference. Zero pressure is larger than negative pressure, and so the pressure outside the seed would be larger than the pressure inside. This would subject the seed to a net external force pressing upon it, much like what your eardrums experience when deep-sea diving. It is the pressure differential is powerful enough to prevent the seed from expanding into the surrounding environment.
But this does not prevent the inflaton's drive to expand. If you blow air into a balloon while tightly clasping its surface, the balloon will bubble out from between your hands. The inflaton seed can behave similarly. The seed can generate a new expanding spatial realm that sprouts from the original spatial environment, as ill.u.s.trated by the little growing sphere in Figure 10.1 Figure 10.1. The calculations show that once the new expanding realm reaches a critical size, its umbilical cord to the parent s.p.a.ce severs, as in the final image of Figure 10.1 Figure 10.1, and an independent inflating universe is born.
As enticing as the process might be-the artificial creation of a new universe-the view from the laboratory wouldn't live up to the advance billing. It's a relief that the inflationary bubble would not gobble up the surrounding environment, but the flip side is that there would be little evidence of the creation itself. A universe that expands by generating new s.p.a.ce, which then detaches from ours, is a universe we can't see. Indeed, as the new universe pinches off, its sole residue would be a deep gravitational well-you can see this in the last image of Figure 10.1 Figure 10.1-which would appear to us as a black hole. And since we have no capacity to see beyond a black hole's edge, we wouldn't even be a.s.sured that our experiment had been a success; without access to the new universe, we would have no means of establis.h.i.+ng observationally that the universe had been created at all.
Figure 10.1 Because of the greater pressure in the ambient environment, an inflationary seed is forced to expand into newly formed s.p.a.ce. As the bubble universe grows, it detaches from the parent environment, yielding a separate, expanding spatial domain. To someone in the ambient environment, the process looks like the formation of a black hole Because of the greater pressure in the ambient environment, an inflationary seed is forced to expand into newly formed s.p.a.ce. As the bubble universe grows, it detaches from the parent environment, yielding a separate, expanding spatial domain. To someone in the ambient environment, the process looks like the formation of a black hole.
Physics protects us, but the price for safety is total separation from our handiwork. And that's the good news.
The bad news for aspiring universe creators is a more sobering result derived by Guth and his MIT colleague Edward Farhi. Their careful mathematical treatment showed that the sequence depicted in Figure 10.1 Figure 10.1 requires an additional ingredient. Much as some balloons require that you give a strong initial burst of air, after which they more easily inflate, Guth and Farhi found that the nascent universe in requires an additional ingredient. Much as some balloons require that you give a strong initial burst of air, after which they more easily inflate, Guth and Farhi found that the nascent universe in Figure 10.1 Figure 10.1 needs a strong kick-start to get the inflationary expansion off and running. So strong that there's only one ent.i.ty that can provide it: a white hole. A white hole, the opposite of a black hole, is a hypothetical object that spews matter out rather than drawing it in. This requires conditions so extreme that known mathematical methods break down (much as is the case at the center of a black hole); suffice it to say, no one antic.i.p.ates generating white holes in the laboratory. Ever. Guth and Farhi found a fundamental wrench in the universe-creation works. needs a strong kick-start to get the inflationary expansion off and running. So strong that there's only one ent.i.ty that can provide it: a white hole. A white hole, the opposite of a black hole, is a hypothetical object that spews matter out rather than drawing it in. This requires conditions so extreme that known mathematical methods break down (much as is the case at the center of a black hole); suffice it to say, no one antic.i.p.ates generating white holes in the laboratory. Ever. Guth and Farhi found a fundamental wrench in the universe-creation works.
A number of research groups have since suggested possible ways of skirting the problem. Guth and Farhi, joined by Jemal Guven, found that by creating the inflationary seed through a quantum tunneling process (similar to what we discussed in the context of the Landscape Multiverse) the white hole singularity can be avoided; but the probability for the quantum tunneling process to happen is so fantastically small that there's essentially no chance of its happening over timescales that anyone would consider worth contemplating. A group of j.a.panese physicists, n.o.buyuki Sakai, Ken-ichi Nakao, Hideki Is.h.i.+hara, and Makoto Kobayas.h.i.+, showed that a magnetic monopole-a hypothetical particle that has either the north pole or the south pole of a standard bar magnet-might catalyze inflationary expansion, also avoiding singularities; but after nearly forty years of intense searching, no one has yet found a single one of these particles.*
As of today, then, the summary is that the door to creating new universes remains open, but only barely. Given the proposals' heavy reliance on hypothetical elements, future developments may well shut this door permanently. But if they don't-or, perhaps, if subsequent work makes a stronger case for the possibility of universe creation-would there be motivation to proceed? Why create a universe if there's no way to see it, or interact with it, or even know for sure that it was was created? Andrei Linde, famous not just for his deep cosmological insights but also for his flair for mock drama, has noted that the allure of playing G.o.d would simply prove irresistible. created? Andrei Linde, famous not just for his deep cosmological insights but also for his flair for mock drama, has noted that the allure of playing G.o.d would simply prove irresistible.
I don't know that it would. Admittedly, it would be thrilling to have so thoroughly grasped nature's laws that we could reenact the most pivotal of all events. I suspect, however, that by the time we can seriously consider universe creation-if that time ever comes-our scientific and technical advancements would have made available so many other spectacular undertakings, whose results we could not just imagine but truly experience, that the intangible nature of universe creation would make it much less interesting.
The appeal would surely be stronger were we to learn how to manufacture universes that we could see or even interact with. For "real" universes, in the usual sense of a universe const.i.tuted from the standard ingredients of s.p.a.ce, time, matter and energy, we don't yet have any strategy for doing so that's compatible with the laws of physics as we currently understand them.
But what if we set aside real universes and consider virtual ones?
The Stuff of Thought.
A couple of years ago, I had a bout of feverish flu that came with hallucinations far more vivid than any ordinary dream or nightmare. In one that has stayed with me, I'd find myself with a group of people sitting in a spa.r.s.e hotel room, locked in a hallucination within the hallucination. I was absolutely certain that days and weeks went by-until I was thrust back into the primary hallucination, where I'd learn, shockingly, that hardly any time had pa.s.sed at all. Each time I felt myself drifting back to the room, I resisted strenuously, since I knew from previous iterations that once there I'd be swallowed whole, unable to recognize the realm as false until I found myself back in the primary hallucination, where I'd again be distraught to learn that what I'd thought real was illusory. Periodically, when the fever subsided, I'd pull out one level further, back to ordinary life, and realize that all those translocations had been taking place within my own swirling mind.
I don't usually learn much from having a fever. But this experience added immediacy to something which, to that point, I'd largely understood only in the abstract. Our grip on reality is more tenuous than day-today life can lead us to believe. Modify normal brain function just a bit, and the bedrock of reality may suddenly s.h.i.+ft; though the outside world remains stable, our perception of it does not. This raises a cla.s.sic philosophical question. Since all of our experiences are filtered and a.n.a.lyzed by our respective brains, how sure are we that our experiences reflect what's real? In the framing philosophers like to use: How do you know you're reading this sentence, and not floating in a vat on a distant planet, with alien scientists stimulating your brain to produce the thoughts and experiences you deem real?
These issues are central to epistemology, a philosophical subfield that asks what const.i.tutes knowledge, how we acquire it, and how sure we are that we have it. Popular culture has brought these scholarly pursuits to a wide audience in films such as The Matrix, The Thirteenth Floor The Matrix, The Thirteenth Floor, and Vanilla Sky Vanilla Sky, tussling with them in entertaining and thought-provoking ways. So, in looser language, the question we're asking is: How do you know you're not hooked into the Matrix?
The bottom line is that you can't know for sure. You engage the world through your senses, which stimulate your brain in ways your neural circuitry has evolved to interpret. If someone artificially stimulates your brain so as to elicit electrical crackles exactly like those produced by eating pizza, reading this sentence, or skydiving, the experience will be indistinguishable from the real thing. Experience is dictated by brain processes, not by what activates those processes.
Going a step further, we can consider dispensing with the sloppiness of biological material altogether. Might all your thoughts and experiences be nothing more than a simulation that leverages software and circuitry sufficiently elaborate to mimic ordinary brain function? Are you convinced of the reality of flesh, blood, and the physical world, when actually your experience is only a crowd of electrical impulses firing through a hyper-advanced supercomputer?
An immediate challenge in considering such scenarios is that they easily set off a spiraling skeptical collapse; we wind up trusting nothing, not even our powers of deductive reasoning. My first response to questions like the ones just posed is to work out how much computer power you'd need to stand a chance of simulating a human brain. But if I am indeed part of such a simulation, why should I believe anything I read in neurobiology texts? The books would be simulations too, written by simulated biologists, whose findings would be dictated by the software running the simulation and thus could easily be irrelevant to the workings of "real" brains. The very notion of a "real" brain might itself be computer-generated artifice. Once you can't trust your knowledge base, reality quickly sails to sea.
We'll return to these concerns, but I don't want them to sink us-at least, not yet. So, for the time being, let's drop anchor. Imagine that you are real flesh and blood, and so am I, and that everything you and I take to be real, in the everyday sense of the term, is is real. With all that a.s.sumed, let's take up the question of computers and brainpower. What, roughly, is the processing speed of the human brain, and how does it compare with the capacity of computers? real. With all that a.s.sumed, let's take up the question of computers and brainpower. What, roughly, is the processing speed of the human brain, and how does it compare with the capacity of computers?
Even if we are not stuck in a skeptical mora.s.s, this is a difficult question. Brain function is largely an uncharted territory. But just to get a glimpse of the terrain, however foggy, consider some numbers. The human retina, a thin slab of 100 million neurons that's smaller than a dime and about as thick as a few sheets of paper, is one of the best-studied neuronal cl.u.s.ters. The robotics researcher Hans Moravec has estimated that for a computer-based retinal system to be on a par with that of humans, it would need to execute about a billion operations each second. To scale up from the retina's volume to that of the entire brain requires a factor of roughly 100,000; Moravec suggests that effectively simulating a brain would require a comparable increase in processing power, for a total of about 100 million million (1014) operations per second.4 Independent estimates based on the number of synapses in the brain and their typical firing rates yield processing speeds within a few orders of magnitude of this result, about 10 Independent estimates based on the number of synapses in the brain and their typical firing rates yield processing speeds within a few orders of magnitude of this result, about 1017 operations per second. Although it's difficult to be more precise, this gives a sense of the numbers that come into play. The computer I'm now using has a speed that's about a billion operations per second; today's fastest supercomputers have a peak speed of about 10 operations per second. Although it's difficult to be more precise, this gives a sense of the numbers that come into play. The computer I'm now using has a speed that's about a billion operations per second; today's fastest supercomputers have a peak speed of about 1015 operations per second (a statistic that no doubt will quickly date this book). If we use the faster estimate for brain speed, we find that a hundred million laptops, or a hundred supercomputers, approach the processing power of a human brain. operations per second (a statistic that no doubt will quickly date this book). If we use the faster estimate for brain speed, we find that a hundred million laptops, or a hundred supercomputers, approach the processing power of a human brain.
Such comparisons are likely naive: the mysteries of the brain are manifold, and speed is only one gross measure of function. But most everyone agrees that one day we will have raw computing capacity equal to, and likely far in excess of, what biology has provided. Futurists contend that such technological leaps will yield a world so far beyond familiar experience that we lack the capacity to imagine what it will be like. Invoking an a.n.a.logy with phenomena that lie outside the bounds of our most refined physical theories, they call this visionary roadblock a singularity. One broad-brush prognosis holds that the surpa.s.sing of brainpower by computers will completely blur the boundary between humans and technology. Some antic.i.p.ate a world run rampant with thinking and feeling machines, while those of us still based in old-fas.h.i.+oned biology routinely upload our brain content, safely storing knowledge and personalities in silico in silico, complete with backup drives, for unlimited durations.
This vision may well be hyperbolic. There's little dispute regarding projections of computer power, but the obvious unknown is whether we will ever leverage such power into a radical fusion of mind and machine. It's a modern-day question with ancient roots; we've been thinking about thinking for thousands of years. How is it that the external world generates our internal responses? Is your sensation of color the same as mine? How about your sensations of sound and touch? What exactly is that voice we hear in our heads, the stream of internal chatter we call our conscious selves? Does it derive from purely physical processes? Or does consciousness arise from a layer of reality that transcends the physical? Penetrating thinkers through the ages, Plato and Aristotle, Hobbes and Descartes, Hume and Kant, Kierkegaard and Nietzsche, James and Freud, Wittgenstein and Turing, among countless others, have tried to illuminate (or debunk) processes that animate the mind and create the singular inner life available through introspection.
A great many theories of mind have emerged, differing in ways significant and subtle. We won't need the finer points, but just to get a feel for where the trails have led, here are a few: dualist dualist theories, of which there are many varieties, maintain that there's an essential nonphysical component vital to mind. theories, of which there are many varieties, maintain that there's an essential nonphysical component vital to mind. Physicalist Physicalist theories of mind, of which there are also many varieties, deny this, emphasizing instead that underlying each unique subjective experience is a unique brain state. theories of mind, of which there are also many varieties, deny this, emphasizing instead that underlying each unique subjective experience is a unique brain state. Functionalist Functionalist theories go further in this direction, suggesting that what really matters to making a mind are the processes and functions-the circuits, their interconnections, their relations.h.i.+ps-and not the particulars of the physical medium within which these processes take place. theories go further in this direction, suggesting that what really matters to making a mind are the processes and functions-the circuits, their interconnections, their relations.h.i.+ps-and not the particulars of the physical medium within which these processes take place.
Physicalists would largely agree that were you to faithfully replicate my brain by whatever means-molecule by molecule, atom by atom-the end product would indeed think and feel as I do. Functionalists would largely agree that were you to focus on higher-level structures-replicating all my brain connections, preserving all brain processes while changing only the physical substrate through which they occur-the same conclusion would hold. Dualists would largely disagree on both counts.
The possibility of artificial sentience clearly relies on a functionalist viewpoint. A central a.s.sumption of this perspective is that conscious thought is not overlaid on a brain but rather is is the very sensation generated by a particular kind of information processing. Whether that processing happens within a three-pound biological ma.s.s or within the circuits of a computer is irrelevant. The a.s.sumption could be wrong. Maybe a bundle of connections needs a substrate of wrinkled wet matter if it's to gain self-awareness. Maybe you need the actual physical molecules that const.i.tute a brain, not just the processes and connections those molecules facilitate, if conscious thought is to animate the inanimate. Maybe the kinds of information processing that computers carry out will always differ in some essential way from brain functioning, preventing the leap to sentience. Maybe conscious thought is fundamentally nonphysical, as claimed by various traditions, and so lies permanently beyond the reach of technological innovation. the very sensation generated by a particular kind of information processing. Whether that processing happens within a three-pound biological ma.s.s or within the circuits of a computer is irrelevant. The a.s.sumption could be wrong. Maybe a bundle of connections needs a substrate of wrinkled wet matter if it's to gain self-awareness. Maybe you need the actual physical molecules that const.i.tute a brain, not just the processes and connections those molecules facilitate, if conscious thought is to animate the inanimate. Maybe the kinds of information processing that computers carry out will always differ in some essential way from brain functioning, preventing the leap to sentience. Maybe conscious thought is fundamentally nonphysical, as claimed by various traditions, and so lies permanently beyond the reach of technological innovation.
With the rise of ever more sophisticated technologies, the questions have become sharper and the pathway toward answers more tangible. A number of research groups have already taken the initial steps toward simulating a biological brain on a computer. For example, the Blue Brain Project, a joint venture between IBM and the ecole Polytechnique Federale in Lausanne, Switzerland, is dedicated to modeling brain function on IBM's fastest supercomputer. Blue Gene, as the supercomputer is called, is a more powerful version of Deep Blue, the computer that triumphed in 1997 over the world chess champion Garry Kasparov. Blue Brain's approach is not all that different from the scenarios I just described. Through painstaking anatomical studies of real brains, researchers are gathering ever more precise insight into the cellular, genetic, and molecular structure of neurons and their interconnections. The project aims to encode such understanding, for now mostly at the cellular level, in digital models simulated by the Blue Gene computer. To date, researchers have drawn on results from tens of thousands of experiments focused on a pinhead-sized section of a rat brain, the neocortical column, to develop a three-dimensional computer simulation of roughly 10,000 neurons communicating through some 10 million interconnections. Comparisons between the response of a real rat's neocortical column and the computer simulation to the same stimuli show an encouraging fidelity of the synthetic model. This is far from the 100 billion neurons firing away in a typical human head, but the project's leader, the neuroscientist Henry Markram, antic.i.p.ates that before 2020 the Blue Brain Project, leveraging processing speeds that are projected to increase by a factor of more than a million, will achieve a full simulated model of the human brain. Blue Brain's goal is not to produce artificial sentience, but rather to have a new investigative tool for developing treatments for various forms of mental illness; still, Markram has gone out on a limb to speculate that, when completed, Blue Brain may very well have the capacity to speak and to feel.
Regardless of the outcome, such hands-on explorations are pivotal to our theories of mind; I'm quite certain that the issue of which, if any, of the competing perspectives are on target cannot be settled through purely hypothetical speculation. In practice, too, challenges are immediately evident. Suppose a computer one day professes to be sentient-how would we know whether it really is? I can't even verify such claims of sentience when made by my wife. Nor she with me. That's a burden arising from consciousness being a private affair. But because our human interactions yield abundant circ.u.mstantial evidence supporting the sentience of others, solipsism quickly becomes absurd. Computer interactions may one day reach a similar point. Conversing with computers, consoling and cajoling them, may one day convince us that the simplest explanation for their apparent conscious self-awareness is that they are indeed conscious and self-aware.
Let's take a functionalist viewpoint, and see where it leads.
Simulated Universes.
If we ever create computer-based sentience, some would likely implant the thinking machines in artificial human bodies, creating a mechanical species-robots-that would be integrated into conventional reality. But my interest here is in those who would be drawn by the purity of electrical impulses to program simulated environments populated by simulated beings that would exist within a computer's hardware; instead of C-3PO or Data, think Sims or Second Life, but with inhabitants who have self-aware and responsive minds. The history of technological innovation suggests that iteration by iteration, the simulations would gain verisimilitude, allowing the physical and experiential characteristics of the artificial worlds to reach convincing levels of nuance and realism. Whoever was running a given simulation would decide whether the simulated beings knew that they existed within a computer; simulated humans who surmised that their world was an elaborate computer program might find themselves taken away by simulated technicians in white coats and confined to simulated locked wards. But probably the vast majority of simulated beings would consider the possibility that they're in a computer simulation too silly to warrant attention.
You may well be having that very reaction right now. Even if you accept the possibility of artificial sentience, you may be persuaded that the overwhelming complexity of simulating an entire civilization, or just a smaller community, renders such feats beyond computational reach. On this point, it's worth looking at some more numbers. Our distant descendants will likely fas.h.i.+on ever-larger quant.i.ties of matter into vast computing networks. So allow imagination free rein. Think big. Scientists have estimated that a present-day high-speed computer the size of the earth could perform anywhere from 1033 to 10 to 1042 operations per second. By comparison, if we a.s.sume that our earlier estimate of 10 operations per second. By comparison, if we a.s.sume that our earlier estimate of 1017 operations per second for a human brain is on target, then an average brain performs about 10 operations per second for a human brain is on target, then an average brain performs about
The Hidden Reality Part 10
You're reading novel The Hidden Reality Part 10 online at LightNovelFree.com. You can use the follow function to bookmark your favorite novel ( Only for registered users ). If you find any errors ( broken links, can't load photos, etc.. ), Please let us know so we can fix it as soon as possible. And when you start a conversation or debate about a certain topic with other people, please do not offend them just because you don't like their opinions.
The Hidden Reality Part 10 summary
You're reading The Hidden Reality Part 10. This novel has been translated by Updating. Author: Brian Greene already has 769 views.
It's great if you read and follow any novel on our website. We promise you that we'll bring you the latest, hottest novel everyday and FREE.
LightNovelFree.com is a most smartest website for reading novel online, it can automatic resize images to fit your pc screen, even on your mobile. Experience now by using your smartphone and access to LightNovelFree.com
- Related chapter:
- The Hidden Reality Part 9
- The Hidden Reality Part 11
RECENTLY UPDATED NOVEL

Leveling Up And Becoming Undefeatable
Leveling Up And Becoming Undefeatable Chapter 1128: Game Bug View : 4,318,328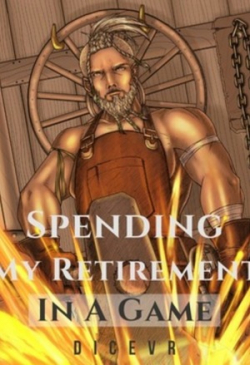
Spending My Retirement In A Game
Spending My Retirement In A Game Chapter 916 True Intentions View : 1,189,153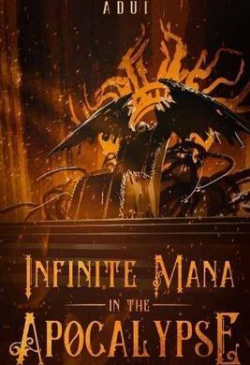
Infinite Mana In The Apocalypse
Infinite Mana In The Apocalypse Chapter 3450 A Spark! IV View : 4,824,713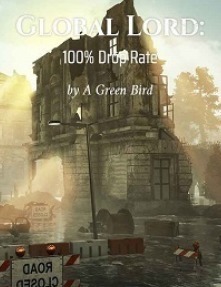
Global Lord: 100% Drop Rate
Global Lord: 100% Drop Rate Chapter 1719 The Mystery Of The Ultimate Void Battlefield! (1) View : 1,044,153
I Love Destroying Worlds' Plot
I Love Destroying Worlds' Plot Chapter 1435 19.94 Second Red Star - Path [End] View : 480,541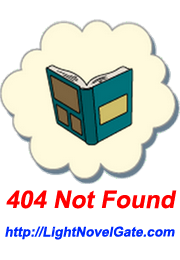