The Party's Over Part 7
You’re reading novel The Party's Over Part 7 online at LightNovelFree.com. Please use the follow button to get notification about the latest chapter next time when you visit LightNovelFree.com. Use F11 button to read novel in full-screen(PC only). Drop by anytime you want to read free – fast – latest novel. It’s great if you could leave a comment, share your opinion about the new chapters, new novel with others on the internet. We’ll do our best to bring you the finest, latest novel everyday. Enjoy!
Geothermal power - whether used for heating or for electricity generation - is necessarily dependent upon geography: plants must be located close to hot springs, geysers, and fumaroles (holes near volcanoes from which vapor escapes). Most geothermal resources are located around the edges of tectonic plates. The west coasts of the Americas as well as Iceland, India, Kenya, the Philippines, Indonesia, j.a.pan, and Thailand all have exploitable geothermal resources.
The US currently has 44 percent of the world's developed geothermal-electric capacity, but the American geothermal industry is stagnant. Less than one percent of the world's electricity production comes from geothermal sources.
By Odum's calculations, geothermal electricity production may currently have an EROEI even higher than that of petroleum (though still far below oil's net yield through the 1960s). However, many geographic locations do not permit the attainment of this degree of net-energy return for geothermal electricity. Moreover, it is unlikely that the generation of electricity from geothermal sources can be increased sufficiently to offset much of the net-energy decline from petroleum depletion.
There is some debate as to whether geothermal electricity production actually const.i.tutes a renewable energy source. As underground steam or hot water is used to turn turbines, it is gradually depleted. The period in which depletion reaches the point where the resource is no longer commercially useful is estimated to be in the range of 40 to 100 years for most geothermal fields. While fields may naturally recharge themselves over a period of centuries or millennia, that will be of little benefit to the next few generations. At The Geysers fields in northern California, efforts are being made to recharge underground reservoirs with treated waste water pumped from the city of Santa Rosa; however, it is too early to tell what the results will be. If successful, the scheme could make geothermal energy production renewable, though the infrastructure and operating costs of the recharging process would drastically reduce the EROEI for energy production from this source.
If recharging efforts fail, the long-term prospects for geothermal electricity look dim. While nations such as Indonesia and Russia have only begun to develop their large potential geothermal resources, without artificial recharging those resources will be useful for only a few decades.
Geothermal energy production has potential for increased local development, but when viewed against the backdrop of the world's total energy needs, its contribution - even if that potential is fully realized - pales in significance.
Tides and Waves.
On the sh.o.r.es of oceans, tides rise and fall predictably day by day. This rising and falling of the tides is a potential source of energy. In a few places, estuaries have been dammed so that water can be let in as the tide rises, and then let out via electricity-generating turbines as the tide falls. For an area with 25-foot tides, Odum calculated an EROEI of 15 - which is the highest net-energy yield for any source he studied. However, this net benefit is substantially reduced when the loss of estuarine fisheries is taken into account.
Tidal energy is renewable, clean, and efficient. Unfortunately, there are fewer than two dozen optimal sites for tidal power in the world, and most of those are in remote areas like northwest Russia or Nova Scotia.
The only US city that is likely to benefit significantly from tidal power is San Francisco, which is committed to developing a one-megawatt tidal power station within two years. A major proponent of this project is HydroVenturi Ltd, whose new technology, developed at Imperial College, has no underwater moving parts. As the tide ebbs and flows, long fins inside an underwater pa.s.sageway would funnel the current, creating suction, which in turn would pull air from pipes connected to onsh.o.r.e turbines, causing the turbines to turn and generate electricity.
If the $2 million test project is a success, it might be possible to power the entire city with electricity generated from the daily tides in the Bay. Potential environmental problems still need to be addressed, including the possibilities that salmon and other fish could be caught in the fins by sudden drops in water pressure; that alteration of the tidal flow could have a negative impact on other marine life; or that increased sediment buildup in the Bay could impair water quality.
Meanwhile a Canadian company, Blue Energy, has created and marketed a highly efficient underwater vertical-axis windmill that can be used to generate tidal power for almost any coastal community. Blue Energy's scalable technology (from a few kilowatts to thousands of megawatts) is claimed to generate efficient, renewable, and emission-free electricity at prices compet.i.tive with today's conventional sources of energy. The design of the turbine is structurally and mechanically straightforward, and the transmission and electrical systems are similar to existing hydroelectric installations.34 There is also tremendous energy inherent in the waves that constantly lap the ocean sh.o.r.es, and it is theoretically possible to harness some of that energy. But doing so is difficult. Waves are extremely variable: they can occasionally reach 60 feet in height, but days or weeks may go by when the ocean is calm. In j.a.pan, Norway, Denmark, Britain, Belgium, and India, a variety of systems have been used to tap wave energy. The results have been mixed: energy has been produced at relatively low cost, but it tends to be intermittently available. A comprehensive survey of wave-energy research by David Ross suggests that this source can provide only limited power for industrial societies for the foreseeable future.35 Bioma.s.s, Biodiesel, and Ethanol.
"Bioma.s.s" is a modern term for what is, in fact, our oldest fuel source: plant material. Current and potential forms of bioma.s.s include wood, animal waste, seaweed, peat, agricultural waste such as sugar cane or corn stalks, and garbage.
As noted in Chapter 2, wood was the princ.i.p.al energy source in the US until the latter part of the 19th century, and it still is in many parts of the world. Deforestation in places like Bangladesh and Haiti is directly attributable to the overharvesting of trees for fuel. In the US, bioma.s.s provides more total energy than hydroelectric power, making it the nation's princ.i.p.al renewable energy source (though hydro is its foremost renewable source for electricity production).
Bioma.s.s has an extremely variable EROEI. However, the burning of all forms of bioma.s.s creates air pollution, which can sometimes be severe. Burning wood for heat releases not only carbon dioxide but a c.o.c.ktail of toxic substances including nitrogen oxides, carbon monoxide, organic gases, and particulate matter. In India, 200 million tons of cow dung are burned annually as cooking fuel; the practice deprives the soil of needed nutrients and also blankets cities in a pollutant haze.
There is limited growth potential for total energy from bioma.s.s. Many parts of the world already are experiencing severe and growing shortages of firewood - which is so scarce in parts of Colombia, Peru, India, Pakistan, Bangladesh, Nepal, and some countries of Africa that many people are reduced to having only one cooked meal a day.
In addition to directly burning bioma.s.s for heat or light, it is also possible to make fuels from it to run machinery and vehicles. When Rudolf Diesel invented the diesel engine in the late 1890s, he envisioned it running on a variety of fuels, including peanut oil. Today's diesel fuel is a refined petroleum product, but diesel engines can still be modified to run on vegetable oils.
Unmodified diesel engines can burn a fuel known as "biodiesel," which is a chemically altered vegetable oil. The production process for the latter is fairly simple: aside from vegetable oil, the two main ingredients are methanol and lye, and with a little practice and some basic equipment it is possible to produce batches of low-cost biodiesel in one's garage using discarded restaurant deep-fry cooking oil.
Personally, I love biodiesel; I run my car on it. Biodiesel has some distinct advantages over petroleum-based diesel fuel. When burned, it produces fewer pollutants - significantly less CO2, less particulate matter, no aromatics (benzene, toluemene, xylene), and no sulfur, though nitrogen oxide emissions are the same as with conventional diesel fuel. Mileage per gallon is typically slightly less for biodiesel than for conventional diesel fuel, but users of the former report that the exhaust from their cars or trucks tends pleasantly to smell like French fries or donuts (depending on the oil source).
However, for all its advantages, biodiesel may be destined to remain merely a "boutique" fuel: currently, there are fewer than ten biodiesel plants in the US and only 21 retail pumps scattered throughout the country; moreover, commercial biodiesel sells for over $3 per gallon - significantly more than conventional diesel fuel. An even worse problem is that the production of vegetable oil for use as a fuel is usually, depending on the type of oil, a net energy loser. The National Renewable Energy Laboratory has performed experiments with the extraction of oil from algae, showing that this source could be extremely productive - several times more so than palms or coconuts. However, it has not been shown that this procedure can be scaled up to produce significant commercial quant.i.ties of oil. Given the petroleum-intensive nature of modern agriculture, it probably takes more energy to produce a gallon of biodiesel than the biodiesel yields when burned; but if further research on algae oil continues to yield promising results, it is possible that a favorable net-energy production could be achieved and a sizeable portion of the diesel fleet could be run on biofuels.
While most enthusiasts use vegetable oil in the form of biodiesel, some modify their diesel car's fuel system to accept ordinary, recycled vegetable oil. Both strategies appeal to a tiny but growing number of environmentally aware motorists who have started fuel-sharing co-ops and who maintain websites devoted to the promotion of vegetable oil-fueled transportation. While there simply aren't enough fast-food restaurants or donut shops to fuel large fleets of cars and trucks, this is a good option for the few mavericks willing to make the effort.
Ethanol - a fuel-grade form of alcohol produced from grain fermentation - suffers from the net-energy constraints similar to those of biodiesel. Promoters tout ethanol as a clean energy alternative since it produces fewer pollutants when burned than do petroleum byproducts, and the US Congress has adopted laws requiring ethanol to be mixed with gasoline for automobile consumption. Essentially, this Federal mandate amounts to a subsidy for agribusiness, since ethanol is produced primarily from corn grown in the American Midwest. Altogether, the ethanol industry receives about $1.4 billion per year in direct or indirect subsidies, most of which end up benefiting giant agribusiness cartels such as Archer Daniels Midland.
Cornell University professor David Pimentel, who has performed two net-energy a.n.a.lyses of ethanol, found in both instances that the fuel cost more energy to produce than it eventually delivered to society. While his recent study was more favorable than the previous one, it nevertheless showed an EROEI of roughly 0.81, meaning a 29 percent net loss of energy.36 However, Pimentel's studies have been attacked by ethanol proponents, who cite much more favorable reports - especially several USDA studies led by Hosein Shapouri, the most recent of which comes to the optimistic conclusion that ethanol offers up to a 77 percent energy profit.37 But Shapouri's and other ethanol-favorable studies have in turn been devastatingly critiqued by Tad W. Patzek of University of California, Berkeley, in a 114 page paper t.i.tled "Thermodynamics of Corn-Ethanol Biofuel Cycle."38 Patzek argues that Shapouri has disregarded or minimized several important energy inputs to the process of ethanol production; once these figures are corrected, the net energy gain cited in the USDA studies is "insupportable."
Ethanol proponents correctly point out that crops other than corn (such as sugar cane) can yield more alcohol per acre; moreover, engines that burn ethanol may last considerably longer than gasoline-burning engines, thus leading to energy savings elsewhere in the industrial system.
Proponents also point to Brazil's experimental use of ethanol from sugar cane as a vehicle fuel in the 1980s. An impressive 91 percent of Brazilian cars produced in 1985 ran on sugar-cane ethanol. However, as world oil prices plummeted during the latter half of the decade, and as sugar prices rose, demand for alcohol-fueled cars subsided. It could be argued that Brazil was able to afford its ethanol experiment primarily because of its favorable ratio of available cropland to automobiles: even if energy and topsoil were being lost in the exercise, the country was temporarily able to absorb these losses because they were small and temporary; the situation would likely be very different in the US.
Brazil remains the world's largest producer of ethyl alcohol, supplying 38 percent of the worldwide total. Yet many environmentalists have expressed fears that if demand for ethanol accelerates, Brazil could be transformed into one giant sugar cane field. Already Brazilian agriculture is encroaching on the cerrado, a vast gra.s.sland and savannah region in the southeastern section of the central Brazilian plateau const.i.tuting a unique and seriously threatened ecosystem.
If the US were to attempt to imitate Brazil's feat, how much farmland would be needed to provide enough ethanol to replace fossil fuels? The United States has about 400 million acres of cropland and about 200 million cars. American farmers produce about 7,110 pounds of corn per acre per year, and an acre of corn yields about 341 gallons of ethanol. The typical American driver would burn 852 gallons of ethanol per year, thus requiring 2.5 acres of cropland. According to this calculation, 500 million acres of farmland would be needed to provide fuel for the American fleet - or 25 percent more farmland than currently exists. (This a.s.sumes that no farmland would be used to grow food.) While ethanol advocates point out that land used for ethanol production can simultaneously be used to produce cattle feed (which is how corn is mostly used these days anyway), the above calculation should nevertheless give us pause, especially given the fact that in 2005 the US will become a net food importer (in dollar terms) for the first time in its history as a nation.39 Even if we accept the controversial claim that ethanol can be produced in such a way as to yield a net energy profit, it would be foolish to a.s.sume that a large percentage of US fleet could be run on the fuel, given the above environmental constraints. If our goal is a sustainable energy regime, it is more realistic merely to envision organic farmers devoting a portion of their land to the production of modest amounts of ethanol with which to run their farm machinery.
Fusion, Cold Fusion, and Free-Energy Devices.
Some people maintain that there are energy sources not constrained by the laws of physics as presently understood. It would be simple enough to write off this viewpoint as pseudoscience; however, in the context of the resource depletion discussion, such claims deserve to be addressed. Are free-energy devices possible?
In essence, a free-energy (or "over-unity") device is one that produces more power than it consumes in its operation. The search for free energy (formerly referred to as "perpetual motion") began long ago. In the 14th century, Villand de Honnecourt produced a drawing of a perpetual-motion machine, as did Leonardo da Vinci a couple of centuries later. Johanes Taisnerius, a Jesuit priest, worked on a perpetual-motion machine based on permanent magnets; and Cornelis Drebbel, an alchemist and magician, supposedly made one in 1610. The first English perpetual-motion patent was granted in 1635; by 1903, 600 such patents had been granted. In the 19th century, so many people were working on perpetual-motion machines that their goal inspired a musical genre - the perpetuum mobile - which transfixed the audiences of virtuosi like Nicolo Paganini and Carl Maria von Weber.
In the 20th century, the free-energy literature tended to center on the work of Nikola Tesla (whose career is briefly discussed in Chapter 2). Tesla produced most of his useful inventions before 1910; thereafter his work became progressively more obscure - some would say, visionary. According to one often repeated (and likely apocryphal) anecdote, in 1931 the reclusive inventor fitted a new Pierce-Arrow with a mysterious 80-horsepower, alternating-current electric motor that had no batteries and no external power source, and drove it for a week.
Unlike Tesla, most 20th-century claimants to perpetual motion were relatively obscure figures. In the 1920s, a self-taught inventor named Lester Hendershot built a generator comprising twin basket-weave coils, capacitors, transformers, and an input magnet/clapper unit, which reputedly produced useful electrical power at about 300 watts. The device tended to be erratic, as its operation depended on the tuning of the input component; moreover, Hendershot himself was unable to provide a scientific explanation of how the device worked.
Also in the 1920s, Dr. T. Henry Moray of Salt Lake City began experimenting with solid-state circuitry, cold cathode-ray tubes, and a radiant-energy device that produced up to 50 kilowatts of electrical power. Similar radiant-energy devices were developed independently by L. R. Crump, Peter Markovitch, and others. Several patents were granted, and efforts have more recently been made to explain the phenomenon in terms of "neutrino flux" and "tachyon fields."
In the 1980s, an inventor named Joseph Newman introduced a series of machine generators built around a powerful permanent magnet rotating within a coil consisting of a very large number of turns of copper wire. In the course of his promotional efforts, Newman gave a weeklong demonstration in the Super Dome in New Orleans and appeared on the Tonight show. He claimed that his machine produced much more energy than it consumed, but critics maintained that the apparent surplus of power (most of which was dissipated in heat) was actually the result of measurement errors.
The above in no way const.i.tutes an exhaustive list of perpetual-motion or free-energy claimants. There are and have been literally scores of others - some deliberate hoaxers, others sincere but naive backyard tinkerers, and still others serious scientists. Many of their efforts seem transparently quixotic. Their goal is clear enough: If only we can find a new, infinite source of energy, we can free ourselves from all sorts of material constraints. But how do free-energy researchers explain - to themselves or their investors - that such a thing is even possible?
The standard textbook view of energy begins with the First Law of Thermodynamics, which states that the sum of all matter and energy in the universe is constant and that energy can be neither created nor destroyed - only its form changes. There are no exceptions: this is the most fundamental law by which we must live, one that cannot be bent, broken, or repealed. What makes free-energy advocates think they can get around it? Is there a loophole?
The best chance of finding one, some suggest, is by way of quantum physics. Theoretical physicists speculate that empty s.p.a.ce may not really be empty after all; it may, in fact, be filled with energy. If so, all we would need to do to harvest that energy would be to a.s.semble the equivalent of a quantum windmill to capture the quantum "wind."
If the details of the process are a bit abstruse, the fact that the well-known science fiction writer Arthur C. Clarke has endorsed the possibility of obtaining energy from vacuum - this is sometimes called "zero-point" energy - is encouraging. Perhaps the search for new energy sources has outgrown the garages of inventors like Hendershot and is ready to move into university physics labs.
Another potential path toward free energy is cold fusion. In 1989, physicists Stanley Pons and Martin Fleishman of Salt Lake City announced that they had produced a nuclear-fusion reaction at room temperature - a feat previously considered impossible. Cold fusion reputedly occurs when ordinary hydrogen and an isotope of hydrogen called deuterium are brought together with metals such as palladium, t.i.tanium, and lithium. The reaction (again, reputedly) releases enormous quant.i.ties of energy - more than ordinary chemical reactions could possibly yield. Cold fusion, in contrast to hot fusion, happens in a relatively simple apparatus roughly the size of a postage stamp and does not emit neutron radiation. It also gives off very little, if any, of the radiation common to nuclear-fission reactions.
Many American scientists still consider cold fusion a form of crank science, though well over 1,000 peer-reviewed papers on the subject have been published. Cold-fusion researchers have never claimed that the effect produces power from vacuum or that it violates any known laws of physics.40 What impact will any of these efforts to develop exotic energy devices have on the energy shortages of the 21st century? In the near term, very little. In all likelihood, most if not all of the ballyhooed free-energy claims of the past were the result of deliberate deception, measurement error, or naivete on the part of unschooled researchers. Moreover, it is difficult to avoid the impression that many of the current Internet discussions of exotic energy devices are pervaded by paranoia and extravagant claims, such as, "The oil companies are buying up all the patents and suppressing the evidence!" or "A secret, unelected military government is running free-energy 'black' projects with technology stolen from s.p.a.ce aliens!" or, "Our only hope is to quickly fund this or that maverick inventor, whose latest device generates a million times more power than it consumes!"
Is the US government really secretly experimenting with free-energy devices? That is entirely possible. It is even possible (in the sense that almost anything is possible) that the technology was acquired from s.p.a.ce aliens. The problem with discussing the subject is that most secret government programs are surrounded with disinformation spread by well-paid experts. Given the continual rain of lies and half-truths about military or intelligence "black" projects, it is impossible to know what to believe about them, and under such circ.u.mstances most speculation is a waste of time.
Sensationalism aside, it appears that, even if cold-fusion devices or "quantum windmills" could work, harnessing these new power sources would not be easy. Currently, the world derives exactly zero percent of its commercially produced energy from all of these exotic sources combined. It is likely that, even in the best case, decades of further research and development would be required to change that statistic appreciably.
Energy production from conventional or hot nuclear fusion is less controversial from a theoretical point of view than are proposed zero-point or cold-fusion projects. Billions of research dollars have been devoted to fusion research over the past two decades. If made practical, fusion could produce almost limitless energy from seawater. However, the hurdles to actually producing fusion energy are prodigious. Reactor temperatures would have to be in the range of 360 million degrees Fahrenheit (200 million degrees Celsius), and no materials or processes are currently capable of containing such temperatures for more than a tiny fraction of a second. No fusion reactor has yet succeeded in producing more energy than it consumes. Even promoters say that commercially useful power production from fusion is at least 50 years away - but it may not be possible to continue funding expensive and energy-intensive fusion research in the energy-constrained environment of the 21st century.
Conservation: Efficiency and Curtailment.
Nearly everyone agrees that the best ways to cus.h.i.+on the impact of an energy shortage are simply to consume less and to get the most out of what we do use. The term conservation is often employed to refer to these two parallel but fundamentally different strategies. The first strategy - perhaps more accurately termed curtailment - would, for example, translate into the prosaic action of turning off a light when leaving a room. The second - more accurately termed efficiency - would, in terms of the same example, mean replacing an incandescent bulb with a compact fluorescent bulb that produces the same amount of light from a quarter of the electricity. There is plenty of room for energy savings from both strategies.
In the past three decades, American homes and workplaces have become much more energy efficient. In the 1950s, the US economy as a whole used over 20,000 BTU for every inflation-adjusted dollar of gross domestic product; by 2000, it was consuming only about 12,000 BTU per dollar. Much of this improvement in efficiency was due to the redesign of common appliances such as refrigerators, lamps, and was.h.i.+ng machines. Today's houses are typically insulated better than houses a few decades ago, and most buildings and factories have been redesigned for energy efficiency.
More such gains are possible. Between 1980 and 1995 the fuel efficiency of US automobiles improved dramatically, but since then that trend has reversed. Cars themselves did not become less efficient; instead, many drivers - encouraged by low gas prices - began buying light trucks or sport utility vehicles, which typically use much more fuel than smaller cars. Toyota and Honda have begun marketing hybrid gasoline-electric cars that achieve over 50 miles per gallon, and American automakers are beginning to roll out their own hybrid versions of existing cars - including SUVs. In the future, an 80 mpg full-size car is probably feasible. Many homes can still benefit greatly from extra insulation, low-e windows, the planting of shade trees to reduce the need for air conditioning, and the replacement of incandescent bulbs with compact fluorescents.
Substantially increased energy savings from efficiency are also possible in industry. Philips, a large European manufacturing firm, is a typical success story in this regard. After deciding in the early 1990s to target energy efficiency, the company hired consultants and began making changes in its operations. Between 1994 and 1999, Philips improved energy efficiency by 31 percent, while reducing its waste stream by 56 percent.
The efficiency of US electricity generation plants peaked in 1958 at about 35 percent. However, newer plant designs are able to achieve efficiencies of 57 percent or more. In 1998, two-thirds of electric generating plants were more than 25 years old; replacing half of these with new, more efficient plants could increase available electricity by about 25 percent with no increase in fossil fuel consumption. Moreover, waste heat from generating plants could be employed to heat homes and factories, or to raise the efficiency of hydrogen production.
Many of these potential improvements could be speeded up through offering subsidies or tax incentives, and energy markets could benefit greatly from intelligent regulations that promote efficient energy provision and consumption. Such an intelligent redesign of regulations in the UK in the 1990s led to a significant increase in energy efficiency, a decrease in the use of nuclear power, and a 39-percent reduction in CO2/kWh.
However, there are limits to the benefits from efficiency, since increasing investments in energy efficiency typically yield diminis.h.i.+ng returns. Initial improvements tend to be easy and cheap; later ones are more costly. Also, the energy costs of retooling or replacing equipment and infrastructure can sometimes wipe out gains. A simple example: Suppose you are currently driving a two-year-old car that travels 25 miles on a gallon of gasoline. You see a similar new car advertised that gets 30 mpg It would appear that, by trading cars, you would be conserving energy. However, the situation is not that simple, since a little over ten percent of all the energy consumption attributable to each vehicle on the road occurs in the manufacturing process - before that vehicle has traveled its first mile. Thus, by putting off trading cars you might be conserving more net energy than you would be by buying the new, more fuel-efficient replacement.
In the late 1980s, Gever et al. studied the relations.h.i.+p between energy efficiency and national economies, as expressed in the ratio between gross domestic product (GDP) and total energy consumed (a rising ratio of GDP to energy consumption means that the economy is becoming more energy-efficient). Not surprisingly, they found that nations like Sweden, Switzerland, and Denmark were much more energy-efficient than the US, and that US energy efficiency had improved significantly during the 1980s. But what were the factors driving increased efficiency? Their a.n.a.lysis showed that energy efficiency increases with the use of more energy-dense sources - this is by far the most important factor - as well as with the reduction of household use of energy and with increased energy prices. As industrial nations made the transition from burning coal to using higher net-yield sources - oil, gas, hydro, and nuclear power - energy efficiency improved dramatically. Household energy consumption (which goes mostly to heating homes and fueling cars) does not add as much to the GDP as does industrial use of energy, which goes toward the production of goods and services, and so efficiency improved as households used proportionately less. And higher energy prices encouraged the switch to more energy-stingy technologies. But the authors pointed out that: our a.n.a.lysis indicates that the ability of technical change to increase the goods and services produced from the same amount and mix of fuels is much smaller than most economists claim .... There are several reasons to believe that previous a.s.sessments of technology's ability to save energy were overly optimistic. For one, many a.n.a.lyses ignored important changes in the kinds of fuels used in the economy and in the division of fuel supplies between household and intermediate sectors. As a result, changes in efficiency due to these factors were mistakenly attributed to technological advances and/or fuel prices ....41 The authors also noted that:.
[i]n agriculture, for example, the amount of fuel used directly on a cornfield to grow a kilogram of corn fell 14.6 percent between 1959 and 1970. However, when the calculation includes the fuel used elsewhere in the economy to build the tractors, make the fertilizers and pesticides, and so on, it turns out that the total energy cost of a kilogram of corn actually rose by 3 percent during that period.42 The inescapable implications of these findings are first, that many efforts toward energy efficiency actually const.i.tute a kind of sh.e.l.l game in which direct fuel uses are replaced by indirect ones, usually in the forms of labor and capital, which exact energy costs elsewhere; and second, that the princ.i.p.al factor that enabled industrial countries to increase their energy efficiency in the past few decades - the switch to energy sources of higher net yield - does not const.i.tute a strategy that can be applied indefinitely in the future.
Thus the curtailment of energy usage offers clearer benefits than improved efficiency. By simply driving fewer miles one unequivocally saves energy - regardless of whether one's car is old or new and whether it is more or less efficient.
Some curtailment is painless - as is the case with turning off the lights when one leaves a room or turning down the thermostat at night. But the economy as a whole is inextricably tied to energy usage, and so significant degrees of curtailment throughout society are likely to have noticeable economic consequences.
We have historical data in this regard. In the 1970s and early 1980s, the US curtailed some of its energy usage due to the oil-price shocks of 1973 and 1979. People drove fewer miles in smaller cars and drove more slowly due to lowered speed limits. As a result, the national GDP/energy ratio improved - but at some cost in terms of the standard of living. That cost was relatively easily borne, but that it was indeed a cost is shown by the fact that when fuel prices drifted back downward, people again began driving more and faster, and choosing larger cars.
Given that, from a historical and cross-cultural perspective, Americans' average standard of living is lavish, it would seem that some curtailment of consumption may not be such a bad thing. After all, people currently have to be coaxed and cajoled from cradle to grave by expensive advertising to consume as much as they do. If the message of this incessant propaganda stream were simply reversed, people could probably be persuaded to happily make do with less. Many social scientists claim that our consumptive lifestyle damages communities, families, and individual self-esteem; a national or global ethic of conservation could thus be socially therapeutic.43 However, eventually curtailment means reducing economic activity - it means fewer jobs, goods, and services. It means fundamental changes not only in the pattern of life but also in the quality of life that we have become accustomed to. Mild degrees of curtailment in national energy usage might just involve sacrifices of speed and convenience. Intermediate degrees might imply tradeoffs in health care, transportation, housing s.p.a.ce, and entertainment options. But severe curtailment - unless undertaken systematically over a period of decades - would likely lead to rampant unemployment and shortages of basic necessities.
Energy conservation - both increased efficiency and curtailment of energy usage - will be crucial in cus.h.i.+oning impacts from the depletion of oil. But it is not a panacea.
With such a broad array of alternatives to choose from, many people a.s.sume it must be possible to cobble together a complex strategy to enable a relatively painless transition away from fossil fuels. Surely, for example, by building more wind turbines and fuel cells, by exploiting advances in photovoltaic technologies, and by redoubling our national conservation efforts, we could effortlessly weather the downside of the Hubbert curve.
A recurring subtext of this chapter has been the importance of net-energy a.n.a.lysis. To date, very few such a.n.a.lyses have been performed by impartial and competent parties. It is essential to the welfare of current and future generations that a standardized and well-defined net-energy methodology be adopted by national and international planning agencies. Reliance on market price as a basis for energy policy is shortsighted, because hidden subsidies so often distort the picture. Any standardized EROEI evaluation methodology will inevitably be imperfect, but it will nevertheless provide the public and decision makers alike with much sounder insights into the costs of various energy options before precious resources are committed to them. As we have seen, the net-energy returns for some renewables (particularly wind) already exceed the dwindling returns for nonrenewable coal and domestic petroleum. Other options (such as hydrogen) may lose their l.u.s.ter when looked at closely.
Clearly, we would see the best outcome if all of the nations of the world were to undertake a full-scale effort toward conservation and the transition to renewables, beginning immediately. And undoubtedly some sort of complex strategy will eventually be adopted. But we should not delude ourselves. Any strategy of transition will be costly - in terms of dollars, energy, and/or our standard of living. Odum and Odum summarize the situation succinctly: "Although many energy subst.i.tutions and conservation measures are possible, none in sight now have the quant.i.ty and quality to subst.i.tute for the rich fossil fuels to support the high levels of structure and process of our current civilization."44 This is somewhat of a double message. Renewable alternatives are capable of providing net-energy benefit to industrial societies. We should be investing in them and converting our infrastructure to use them. If there is any solution to industrial societies' approaching energy crises, renewables plus conservation will provide it. Yet in order to achieve a transition from nonrenewables to renewables, decades will be required - and we do not have decades before the peaks in the extraction rates of oil and natural gas occur. Moreover, even in the best case, the transition will require s.h.i.+fting investment from other sectors of the economy (such as the military) toward energy research, conservation, and the implementation of renewable alternatives. Those alternatives will be unable to support the kinds of transportation, food, and dwelling infrastructure we now have; thus the transition will necessarily be comprehensive: it will entail an almost complete redesign of industrial societies. The result - an energy-conserving society that is less mobile, more localized, and more materially modest - may bring highly desirable lifestyle benefits for our descendants. Yet it is misleading to think that we can achieve that result easily or painlessly.
If indeed none of the energy alternatives now available has the near-term potential to "support the high levels of structure and process of our current civilization," then profound changes are virtually inevitable in every sphere of human concern as oil begins to run out. Just what sorts of changes can we expect to see within the next 50 years?
5.
A Banquet of Consequences.
Anyone who believes exponential growth can go on forever in a finite world is either a madman or an economist.
- Kenneth Boulding (ca. 1980).
If we continue ... to consume the world until there's no more to consume, then there's going to come a day, sure as h.e.l.l, when our children or their children or their children's children are going to look back on us - on you and me - and say to themselves, "My G.o.d, what kind of monsters were these people?"
- Daniel Quinn (2000).
Current debates over where and how to drill for oil in this country soon may be rendered irrelevant by a nation desperate to maintain its quality of life and economic productivity. War over access to the diminis.h.i.+ng supply of oil may be inevitable unless the United States and other countries act now to develop alternatives to their dependence on oil.
- Senator Mark Hatfield (1990).
We need an energy bill that encourages consumption.
- George W. Bush (2002).
Sooner or later, we sit down to a banquet of consequences.
- Robert Louis Stevenson (ca. 1885).
When the global peak in oil production is reached, there will still be plenty of petroleum in the ground - as much that will be ultimately recoverable as has been extracted from 1859 to the present, or roughly one trillion barrels (by most estimates). But every year from then on, it will be difficult or impossible to find and pump as much oil as the year before. The rate-curve of extraction necessarily roughly mirrors, with a time lag, the rate-curve of discovery - which peaked in 1964. Even if efforts are intensified now to switch to other energy sources, those efforts will come so late that, for the duration of the transition, society will inevitably have less net energy available to do useful work - including the manufacturing and transporting of goods, the growing of food, and the heating of homes.
During the past two centuries, we have become accustomed to a regime in which there was more energy available each year, and our population has grown quickly to take advantage of this energy windfall. We have come to rely on an economic system built on the a.s.sumption that growth is normal and necessary, and that it can go on forever.
As we move from a historic interval of net-energy growth to one of net-energy decline, we are entering uncharted territory. It takes some effort to adjust one's mental frame of reference to this new reality.
Try the following thought experiment. Go to the center of a city and find a comfortable place to sit. Look around and ask yourself: Where and how is energy being used? What forms of energy are being consumed, and what work is that energy doing? Notice the details of buildings, cars, buses, streetlights, and so on; notice also the activities of the people around you. What kinds of occupations do these people have, and how do they use energy in their work? Try to follow some of the strands of the web of relations.h.i.+ps between energy, jobs, water, food, heating, construction, goods distribution, transportation, and maintenance that together keep the city thriving.
After you have spent at least 20 minutes appreciating energy's role in the life of this city, imagine what the scene you are viewing would look like if there were 10 percent less energy available. What subst.i.tutions would be necessary? What choices would people make? What work would not get done?
Now imagine the scene with 25 percent less energy available; with 50 percent less; with 75 percent less.
a.s.suming that the peak in global oil production occurs at some point in the period from 2006 to 2010 and that there is an average two percent decline in available net energy each year afterward, in your imagination you will have taken a trip into the future, to perhaps the year 2050.
This exercise is, of course, speculative. However, some speculation - if grounded in an awareness of present reality and existing trends - can be helpful.
This chapter consists of speculations about the effects of the net-energy decline during the next few decades. Forecasts are always fallible, as anyone who regularly reads five-day weather forecasts knows. But some scenarios of future events are more likely than others; and with an understanding of how epochs of energy abundance or shortage have shaped the development of societies in the past, we should be able to foresee some of the general outlines of events to come as industrial societies move from a condition of energy abundance to one of energy scarcity.
Clearly, the energy transition of the early 21st century will affect nearly everything that humans care about. No person or group will be untouched by this great watershed.
Because the s.h.i.+ft will be incremental, it would be a mistake to a.s.sume that the effects discussed in this chapter will all occur soon or in an instantaneous fas.h.i.+on. However, it would also be a mistake to a.s.sume that they will be so gradual in their appearance that they will acc.u.mulate to truly dramatic proportions only in our grandchildren's lifetimes or later. The early effects of the net-energy peak are already upon us and will probably begin to cascade within the next two decades or even the next few years.
Industrial civilization is a complexly interrelated ent.i.ty, and it will respond to the net-energy decline as a system. It is therefore problematic to deal separately with effects on agriculture, transportation, and economics because developments in any one area will impact - and be impacted by - developments elsewhere in the system. However, written information must necessarily be organized in a linear fas.h.i.+on, so we will deal with various aspects of society one by one. As we do so, the reader may wish to give some thought to the ways in which each aspect dovetails with the others.
The Economy - Physical and Financial.
The links between the physical economies of nations - their production, distribution, and consumption of goods and services - and the availability of energy are fairly obvious, but the subject bears some discussion nevertheless. All human activities require energy, which physicists define as the capacity to do work. With less net energy available, less work can be done - unless the efficiency of the process of converting energy to work is raised at the same rate as that at which energy availability declines. It will therefore be essential, over the next few decades, for all economic processes to be made as energy-efficient as possible. However, as discussed in the previous chapter, efforts to improve efficiency are subject to diminis.h.i.+ng returns, and so eventually a point will be reached when reduced energy availability will translate into reduced economic activity.
Our current financial system was designed during a period of consistent growth in available energy, with its designers operating under the a.s.sumption that continued economic growth was both inevitable and desirable. This ideology of growth has become embodied in systemic financial structures requiring growth. The most prominent of these is compound interest.
Suppose you were to deposit $100 in a bank account earning six percent interest, and left it there for your children or grandchildren. After the first year, you would have $106, and after the second, $112.30. In twelve years your deposit would have doubled, and in a hundred years it would grow to $33,930. Unfortunately, the compound interest on debt works the same way: if you were to take out a loan of $100 at six percent interest and fail to make payments, over time that debt would grow similarly.
Currently all nations have a type of monetary system in which virtually all money is created through the making of loans. Thus, nearly all of the money in existence represents debt. For those not familiar with banking, this may be a difficult fact to grasp: I find that when I present it to college students, I often have to reiterate it in various ways for an hour or so before they are able to comprehend that money is not a physical substance kept in a vault, but a fict.i.tious ent.i.ty created out of nothing by bankers in order to facilitate the keeping of accounts.
All of this being so, a problem arises: From where does the money come with which to pay back the interest on loans? Ultimately, that money has to come from new loans, taken out by others somewhere else within the financial network of the economy. If new loans are not being made, then somewhere in the network people will be finding it impossible to pay the interest on their existing loans, and bankruptcies will follow. Thus the necessity for growth in the money supply is a structural feature of the financial system. The system seems to function best when growth in the money supply is kept at a low and fairly constant rate, and this is the job of the national banks (in the US, the Federal Reserve; in Canada, the Bank of Canada; in England, the Bank of England, and so on), which adjust interest rates to this end.
If money creation (i.e., the making of loans) occurs more rapidly than the growth in the production and consumption of goods and services in the economy, then inflation results; money then has less purchasing power, and this is bad for lenders - since the money used to repay loans is then worth less than the money that was borrowed. If money is not being loaned out (i.e., created) at a pace fast enough to match the growth in goods and services, then not enough money will be available to repay existing loans (plus interest), and the resulting bankruptcies and foreclosures can - in extreme cases - cause the economy to go into a tailspin of cascading financial cannibalism. Deflation may ensue, in which the purchasing power of money actually increases.
Until now, this loose linkage between a financial system predicated upon the perpetual growth of the money supply and an economy growing year by year because of an increasing availability of energy and other resources has worked reasonably well - with a few notable exceptions, such as the Great Depression. Productivity - the output produced per worker-hour - has grown dramatically, not because workers have worked harder but because workers have been controlling ever more energy in order to accomplish their tasks. Productivity, total economic activity, population, and money supply have all grown - at rates that have fluctuated, but within acceptable ranges.
The lower-energy economy of the future will be characterized by lowered productivity. There could be a good side to this in that more human labor will be required in order to do the same amount of work, with human muscle-power partially replacing the power of fossil fuels. Theoretically, this could translate into near-zero unemployment rates.
However, the financial system may not respond rationally. With less physical economic activity occurring, businesses would be motivated to take out fewer loans. This might predictably trigger a financial crisis, which would in turn likely undermine any attempts at a smooth economic adjustment.
As Hubbert pointed out, the linkage between the money system (the financial economy) and the human matter-energy system (the physical economy) is imperfect. It is possible for a crisis to occur in the financial system even when energy, raw materials, and labor remain abundant, as happened in the 1930s. But is it also possible for the financial system to remain healthy through an energy-led decline in the physical economy? That, unfortunately, is highly unlikely, due to the dependence of the former on continued borrowing to finance activity in the latter. Rather, it is highly likely that the net-energy decline will sooner or later trigger a financial crisis through a reduction in demand for goods and services, and hence for money (via loans) with which to pay for the machinery to produce those goods and services. Thus even if human labor is sufficiently abundant to make up for some of the reduction of work performed by fuel-burning machines, the financial system may not be able to adapt quickly enough to provide employment for potential laborers.
The Party's Over Part 7
You're reading novel The Party's Over Part 7 online at LightNovelFree.com. You can use the follow function to bookmark your favorite novel ( Only for registered users ). If you find any errors ( broken links, can't load photos, etc.. ), Please let us know so we can fix it as soon as possible. And when you start a conversation or debate about a certain topic with other people, please do not offend them just because you don't like their opinions.
The Party's Over Part 7 summary
You're reading The Party's Over Part 7. This novel has been translated by Updating. Author: Richard Heinberg already has 669 views.
It's great if you read and follow any novel on our website. We promise you that we'll bring you the latest, hottest novel everyday and FREE.
LightNovelFree.com is a most smartest website for reading novel online, it can automatic resize images to fit your pc screen, even on your mobile. Experience now by using your smartphone and access to LightNovelFree.com
- Related chapter:
- The Party's Over Part 6
- The Party's Over Part 8
RECENTLY UPDATED NOVEL

A Demon Lord's Tale: Dungeons, Monster Girls, and Heartwarming Bliss
A Demon Lord's Tale: Dungeons, Monster Girls, and Heartwarming Bliss Chapter 564.1 View : 1,340,566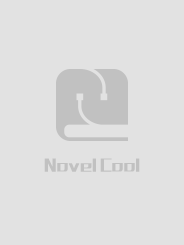
Reborn : Space Intelligent Woman
Reborn : Space Intelligent Woman Chapter 2099: Explain The Situation View : 1,268,128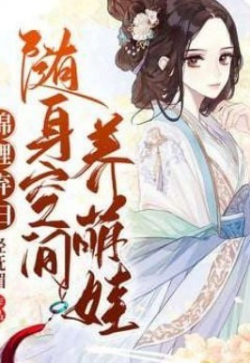
Raising My Children With My Personal Spatial Ability
Raising My Children With My Personal Spatial Ability Chapter 1691: Isn't it easy to capture with one's hands? View : 1,123,116
The Strongest War God
The Strongest War God Chapter 1232: The King's Might Cannot Be Provoked View : 373,180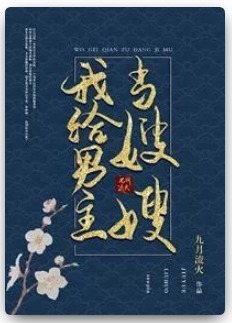
I'll Be the Male Leads Sister-in-Law
I'll Be the Male Leads Sister-in-Law Chapter 535 View : 106,850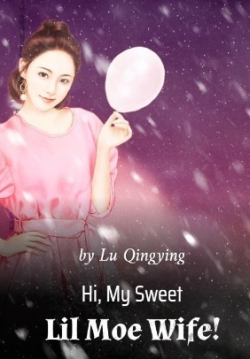
Hi, My Sweet Lil Moe Wife!
Hi, My Sweet Lil Moe Wife! Chapter 2242: Her tyrant is the best View : 414,915