Physics of the Impossible Part 12
You’re reading novel Physics of the Impossible Part 12 online at LightNovelFree.com. Please use the follow button to get notification about the latest chapter next time when you visit LightNovelFree.com. Use F11 button to read novel in full-screen(PC only). Drop by anytime you want to read free – fast – latest novel. It’s great if you could leave a comment, share your opinion about the new chapters, new novel with others on the internet. We’ll do our best to bring you the finest, latest novel everyday. Enjoy!
So Feynman revealed the true secret of antimatter: it's just ordinary matter going backward in time. This simple observation immediately explained the puzzle that all particles have antiparticle partners: it's because all particles can travel backward in time, and hence masquerade as antimatter. (This interpretation is equivalent to the "Dirac sea," mentioned earlier, but it is simpler, and it is the explanation currently accepted today.) Now let's say we have a lump of antimatter and it collides with ordinary matter, creating a huge explosion. There are now trillions of electrons and trillions of antielectrons being annihilated. But if we reversed the direction of the arrow for the antielectron, turning it into an electron going backward in time, this would mean that the same electron went zigzagging backward and forward trillions of times.
There was a further curious result: there must be just one electron in the lump of matter. The same electron went whizzing back and forth, zigzagging in time. Each time it did a U-turn in time it became antimatter. But if it did another U-turn in time then it turned into another electron.
(With his thesis adviser, John Wheeler, Feynman then speculated that perhaps the entire universe consisted of just one electron, zigzagging back and forth in time. Imagine that out of the chaos of the original big bang only a single electron was created. Trillions of years later, this single electron would eventually encounter the cataclysm of Doomsday, where it would make a U-turn and go backward in time, releasing a gamma ray in the process. Then it would go back to the original big bang, and then perform another U-turn. The electron would then make repeated zigzag journeys back and forth, from the big bang to Doomsday. Our universe in the twenty-first century is just a time slice of this electron's journey, in which we see trillions of electrons and antielectrons, that is, the visible universe. As strange as this theory may appear, it would explain a curious fact from the quantum theory: why all electrons are the same. In physics you cannot label electrons. There are no green electrons or Johnny electrons. Electrons have no individuality. You cannot "tag" an electron, like scientists sometimes tag animals in the wild to study them. Maybe the reason is that the entire universe consists of the same electron, just bouncing back and forth in time.) But if antimatter is ordinary matter going back in time, then is it possible to send a message into the past? Is it possible to send today's Wall Street Journal back to yourself in the past, so you can make a killing on the stock market?
The answer is no.
If we treat antimatter as just another exotic form of matter and then perform an experiment with antimatter, there are no violations of causality. Cause and effect remain the same. If we now reverse the arrow of time for the antielectron, sending it backward in time, then we have only performed a mathematical operation. The physics remains the same. Nothing has changed physically. All experimental results remain the same. So it is absolutely valid to view the electron as going backward and forward in time. But each time the electron goes backward in time, it simply fulfills the past. So it appears as if the advanced solutions from the future are indeed necessary to have a consistent quantum theory, but they ultimately do not violate causality. (In fact, without these bizarre advanced waves, causality would be violated in the quantum theory. Feynman showed that if we add the contribution of the advanced and r.e.t.a.r.ded waves, we find that the terms that might violate causality cancel precisely. Thus antimatter is essential to preserving causality. Without antimatter, causality might collapse.) Feynman continued to pursue the germ of this crazy idea until it eventually blossomed into a complete quantum theory of the electron. His creation, quantum electrodynamics (QED), has been experimentally verified to one part in 10 billion, making it one of the most accurate theories of all time. It won him and his colleagues Julian Schwinger and Sin-Itiro Tomonaga the n.o.bel Prize in 1965.
(In Feynman's n.o.bel Prize acceptance speech, he said that as a youth he impulsively fell in love with these advanced waves from the future, like falling in love with a beautiful girl. Today that beautiful girl has matured into a grown woman and is the mother of many children. One of those children is his theory of quantum electrodynamics.) TACHYONS FROM THE FUTURE.
In addition to advanced waves from the future (which have proven their utility over and over again in the quantum theory) there is yet another bizarre concept from the quantum theory that seems just as crazy, but perhaps not as useful. This is the idea of "tachyons," which appear regularly on Star Trek. Anytime the writers of Star Trek need some kind of new energy to perform some magical operation, they invoke tachyons.
Tachyons live in a strange world where everything travels faster than light. As tachyons lose energy, they travel faster, which violates common sense. In fact, if they lose all energy, they travel at infinite velocity. As tachyons gain energy, however, they slow down until they reach the speed of light.
What makes tachyons so strange is that they come with imaginary ma.s.s. (By "imaginary," we mean that their ma.s.s has been multiplied by the square root of minus one, or "i.") If we simply take Einstein's famous equations and replace "m" with "im," then something marvelous happens. All of a sudden particles travel faster than light.
This result gives rise to strange situations. If a tachyon travels through matter, it loses energy because it collides with atoms. But as it loses energy, it speeds up, which further increases its collisions with atoms. These collisions should cause it to lose more energy and hence accelerate even faster. As this creates a vicious cycle, the tachyon naturally attains infinite velocity all by itself!
(Tachyons are different from antimatter and negative matter. Antimatter has positive energy, travels at less than the speed of light, and can be created in our particle accelerators. It falls down under gravity, according to theory. Antimatter corresponds to ordinary matter going backward in time. Negative matter has negative energy and also travels less than the speed of light, but falls up under gravity. Negative matter has never been found in the laboratory. In large quant.i.ties, it can in theory be used to fuel time machines. Tachyons travel faster than light and have imaginary ma.s.s; it's not clear if they fall up or down under gravity. They, too, have not been found in the laboratory.) As bizarre as tachyons are, they have been seriously studied by physicists, including the late Gerald Feinberg of Columbia University and George Sudarshan of the University of Texas at Austin. The problem is that no one has ever seen a tachyon in the laboratory. The key experimental evidence for tachyons would be a violation of causality. Feinberg even suggested that physicists examine a laser beam before it was switched on. If tachyons exist, then perhaps light from the laser beam could be detected even before the apparatus was turned on.
In science fiction stories tachyons are regularly used to send messages back to the past to seers. But if one examines the physics it is not clear if this is possible. Feinberg, for example, believed that the emission of a tachyon going forward in time was identical to the absorption of a negative-energy tachyon going backward in time (similar to the situation with regard to antimatter) and hence there was no violation of causality.
Science fiction aside, today the modern interpretation of tachyons is that they might have existed at the instant of the big bang, violating causality, but they don't exist anymore. In fact, they might have played an essential role in getting the universe to "bang" in the first place. In that sense, tachyons are essential for some theories of the big bang.
Tachyons have a peculiar property. When you put them into any theory, they destabilize the "vacuum," that is, the lowest energy state of a system. If a system has tachyons, it is in a "false vacuum," so the system is unstable and will decay down to the true vacuum.
Think of a dam that holds back the water in a lake. This represents the "false vacuum." Although the dam appears perfectly stable, there is an energy state that is lower than the dam. If a crack develops in the dam and the water comes bursting out of the dam break, the system attains the true vacuum as the water flows toward sea level.
In the same way, the universe before the big bang, it is believed, originally started off in the false vacuum, in which there were tachyons. But the presence of tachyons meant that this was not the lowest energy state, and hence the system was unstable. A tiny "rip" appeared in the fabric of s.p.a.ce-time, representing the true vacuum. As the rip got larger, a bubble emerged. Outside the bubble the tachyons still exist, but inside the bubble the tachyons have all disappeared. As the bubble expands, we find the universe as we know it, without tachyons. This is the big bang.
One theory taken very seriously by cosmologists is that a tachyon, called the "inflation," started the original process of inflation. As we mentioned earlier, the inflationary universe theory states that the universe started off as a tiny bubble of s.p.a.ce-time that underwent a turbocharged inflationary period. Physicists believe that the universe originally started off in the false vacuum state, where the inflation field was a tachyon. But the presence of a tachyon destabilized the vacuum, and tiny bubbles formed. Inside one of these bubbles the inflation field a.s.sumed the true vacuum state. This bubble then began to inflate rapidly, until it became our universe. Inside our bubble-universe the inflation has disappeared, so it can no longer be detected in our universe. So tachyons represent a bizarre quantum state in which objects go faster than light and perhaps even violate causality. But they disappeared a long time ago, and perhaps gave birth to the universe itself.
All this may sound like idle speculation that is not testable. But the theory of the false vacuum will get its first experimental test, starting in 2008, when the Large Hadron Collider is turned on outside Geneva, Switzerland. One of the key purposes of the LHC is to find the "Higgs boson," the last particle in the Standard Model, the one that has yet to be found. It is the last piece of this jigsaw puzzle. (The Higgs particle is so important but elusive that n.o.bel laureate Leon Lederman called it "The G.o.d Particle.") The Higgs boson, physicists believe, originally started out as a tachyon. In the false vacuum, none of the subatomic particles had any ma.s.s. But its presence destabilized the vacuum, and the universe made a transition to a new vacuum, in which the Higgs boson turned into an ordinary particle. After the transition from a tachyon to an ordinary particle, the subatomic particles begin to have the ma.s.ses that we measure in the laboratory today. Thus the discovery of the Higgs boson will not only complete the last missing piece of the Standard Model, it will also verify that the tachyon state once existed but has been transformed into an ordinary particle.
In summary, precognition is ruled out by Newtonian physics. The iron rule of cause and effect is never violated. In the quantum theory, new states of matter are possible, such as antimatter, which corresponds to matter going backward in time, but causality is not violated. In fact, in a quantum theory, antimatter is essential to restoring causality. Tachyons at first seem to violate causality, but physicists believe that their true purpose was to set off the big bang and hence they are not observable anymore.
Therefore precognition seems to be ruled out, at least for the foreseeable future, making it a Cla.s.s III impossibility. It would set off a major shake-up in the very foundations of modern physics if precognition was ever proved in reproducible experiments.
Epilogue.
THE FUTURE OF THE IMPOSSIBLE.
There is nothing so big nor so crazy that one out of a million technological societies may not feel itself driven to do, provided it is physically possible.
-FREE MAN DYSON Destiny is not a matter of chance-it is a matter of choice. It is not a thing to be waited for-it is a thing to be achieved.
-WILLIAM JENNINGS BRYAN Are there truths that will be forever beyond our grasp? Are there realms of knowledge that will be outside the capabilities of even an advanced civilization? Of all the technologies a.n.a.lyzed so far, only perpetual motion machines and precognition fall into the category of Cla.s.s III impossibilities. Are there other technologies that are similarly impossible?
Pure mathematics abounds in theorems showing that certain things are truly impossible. One simple example is that it is impossible to trisect an angle using only a compa.s.s and ruler; this was proven back in 1837.
Even in simple systems such as arithmetic there are impossibilities. As I mentioned earlier, it is impossible to prove all the true statements in arithmetic within the postulates of arithmetic. Arithmetic is incomplete. There will always be true statements in arithmetic that can be proven only if one moves to a much larger system that includes arithmetic as a subset.
Although some things in mathematics are impossible, it is always dangerous to declare that something is absolutely impossible in the physical sciences. Let me remind you of a speech given by n.o.bel laureate Albert A. Michelson in 1894 at the dedication of the Ryerson Physical Lab at the University of Chicago, in which he declared that it was impossible to discover any new physics: "The more important fundamental laws and facts of physical science have all been discovered, and these are now so firmly established that the possibility of their ever being supplanted in consequence of new discoveries is exceedingly remote...Our future discoveries must be looked for in the sixth place of decimals."
His remarks were uttered on the eve of some of the greatest upheavals in scientific history, the quantum revolution of 1900, and the relativity revolution of 1905. The point is that things that are impossible today violate the known laws of physics, but the laws of physics, as we know them, can change.
In 1825 the great French philosopher Auguste Comte, writing in Cours de Philosophie, declared that it was impossible for science to determine what the stars were made of. This seemed like a safe bet at the time, since nothing was known about the nature of stars. They were so distant that it was impossible to visit them. Yet just a few years after he made this claim, physicists (using spectroscopy) declared that the sun was made of hydrogen. In fact, we now know that by a.n.a.lyzing the spectral lines from stars emitted billions of years ago it is possible to determine the chemical nature of most of the universe.
Comte challenged the world of science by making a list of other "impossibilities": * He claimed that the "ultimate structure of bodies must always transcend our knowledge." In other words, it was impossible to know the true nature of matter.
* He thought that mathematics could never be used to explain biology and chemistry. It was impossible, he claimed, to reduce these sciences to mathematics.
* He thought that it was impossible that the study of heavenly bodies would have any impact on human affairs.
In the nineteenth century it was reasonable to propose these "impossibilities" since so little was known about fundamental science. Almost nothing was known about the secrets of matter and life. But today we have the atomic theory, which has opened up a whole new realm of scientific investigation into the structure of matter. We know about DNA and the quantum theory, which have unraveled the secrets of life and chemistry. We also know about meteor impacts from s.p.a.ce, which have not only influenced the course of life on Earth, but have helped to shape its very existence.
Astronomer John Barrow notes, "Historians still debate the suggestion that Comte's views were partly responsible for the subsequent decline in French science."
Mathematician David Hilbert, in rejecting Comte's claims, wrote, "The true reason, according to my thinking, why Comte could not find an unsolvable problem lies in the fact that there is no such thing as an unsolvable problem."
But today some scientists are raising a new set of impossibilities: we will never know what happened before the big bang (or why it "banged" in the first place), and we will never achieve a "theory of everything."
Physicist John Wheeler commented on the first "impossible" question when he wrote: "Two hundred years ago, you could ask anybody, 'Can we someday understand how life came into being?' and he would have told you, 'Preposterous! Impossible!' I feel the same way about the question, 'Will we ever understand how the universe came into being?'"
Astronomer John Barrow adds, "The speed at which light travels is limited and so, therefore, is our knowledge of the structure of the Universe. We cannot know whether it is finite or infinite, whether it had a beginning or will have an end, whether the structure of physics is the same everywhere, or whether the Universe is ultimately a tidy or an untidy place...All the great questions about the nature of the Universe-from its beginning to its end-turn out to be unanswerable."
Barrow is correct in saying that we will never know, with absolute certainty, the true nature of the universe, in all its glory. But it is possible to incrementally chip away at these eternal questions and come tantalizingly close. Instead of representing the absolute boundaries of our knowledge, these "impossibilities" may perhaps better be seen as the challenges awaiting the next generation of scientists. These limits are like piecrusts, made to be broken.
DETECTING THE PREBIG BANG ERA In the case of the big bang, a new generation of detectors is being built that could settle some of these eternal questions. Today our radiation detectors in outer s.p.a.ce can only measure the microwave radiation emitted 300,000 years after the big bang, when the first atoms formed. It is impossible to use this microwave radiation to probe earlier than 300,000 years after the big bang, since radiation from the original fireball was too hot and random to yield useful information.
But if we a.n.a.lyze other types of radiation we may be able to get even closer to the big bang. Tracking neutrinos, for example, can take us closer to the instant of the big bang (neutrinos are so elusive that they can travel through an entire solar system made of solid lead). Neutrino radiation could take us within a few seconds after the big bang.
But perhaps the ultimate secret of the big bang will be revealed by examining "gravity waves," waves that move along the fabric of s.p.a.ce-time. As physicist Rocky Kolb of the University of Chicago says, "By measuring the properties of the neutrino background we can look back to one second after the Bang. But gravitational waves from [the] inflation area are relics of the universe 10-35 seconds after the bang."
Gravity waves were first predicted by Einstein in 1916; they may eventually become the most important probe for astronomy. Historically each time a new form of radiation was harnessed, a new era in astronomy was opened up. The first form of radiation was visible light, used by Galileo to investigate the solar system. The second form of radiation was radio waves, which eventually enabled us to probe the centers of galaxies to find black holes. Gravity wave detectors may unveil the very secrets of creation.
In some sense gravity waves have to exist. To see this, consider the age-old question: what happens if the sun suddenly disappears? According to Newton, we would feel the effects immediately. The Earth would be instantly thrown out of its...o...b..t and plunged into darkness. This is because Newton's law of gravity does not take into account velocity, and hence forces act instantly throughout the universe. But according to Einstein, nothing can travel faster than light, so it would take eight minutes for the information about the sun's disappearance to reach the Earth. In other words, a spherical "shock wave" of gravity would emerge from the sun and eventually hit the Earth. Outside this sphere of gravity waves, it would appear as if the sun were still s.h.i.+ning normally, because information about the disappearance of the sun would not have reached Earth. Inside this sphere of gravity waves, however, the sun would have already disappeared, as the expanding shock wave of gravity waves travels at the speed of light.
Another way to see why gravity waves must exist is to visualize a large bed sheet. According to Einstein, s.p.a.ce-time is a fabric that can be warped or stretched, like a curved bed sheet. If we grab a bed sheet and shake it rapidly we see that waves ripple along the surface of the bed sheet and travel at a definite velocity. In the same way, gravity waves can be viewed as waves traveling along the fabric of s.p.a.ce-time.
Gravity waves are among the fastest-moving topics in physics today. In 2003 the first large-scale gravity wave detectors became operational-called LIGO (Laser Interferometer Gravitational Wave Observatory), measuring 2.5 miles in length, one is based in Hanford, Was.h.i.+ngton, and another in Livingston Parish, Louisiana. It is hoped that LIGO, at a cost of $365 million, will be able to detect radiation from colliding neutron stars and black holes.
The next big leap will take place in 2015, when an entirely new generation of satellites will be launched that will a.n.a.lyze gravitational radiation in outer s.p.a.ce from the instant of creation. The three satellites that make up LISA (Laser Interferometer s.p.a.ce Antenna), a joint project of NASA and the European s.p.a.ce Agency, will be sent into orbit around the sun. These satellites will be capable of detecting gravitational waves emitted less than a trillionth of a second after the big bang. If a gravity wave from the big bang still circulating around the universe hits one of the satellites, it will disturb the laser beams, and this disturbance can then be measured in a precise way, giving us "baby pictures" of the instant of creation itself.
LISA consists of three satellites circling the sun arranged in a triangle, each connected by laser beams 3 million miles long, making it the largest instrument of science ever created. This system of three satellites will orbit the sun about 30 million miles from the Earth.
Each satellite will emit a laser beam with only half a watt of power. By comparing the laser beams coming from the other two satellites, each satellite will be able to construct an interference pattern of light. If a gravity wave disturbs the laser beams, it will change the interference pattern, and the satellite will be able to detect this disturbance. (The gravity wave does not make the satellites vibrate. It actually creates a distortion in the s.p.a.ce between the three satellites.) Although the laser beams are very weak, their accuracy will be astounding. They will be able to detect vibrations to within one part in a billion trillion, corresponding to a s.h.i.+ft 1/100 the size of an atom. Each laser beam will be able to detect a gravity wave from a distance of 9 billion light-years, which covers most of the visible universe.
LISA has the sensitivity to potentially differentiate between several "prebig bang" scenarios. One of the hottest topics in theoretical physics today is calculating the characteristics of the prebig bang universe. At present, inflation can describe quite well how the universe evolved once the big bang took place. But inflation cannot explain why the big bang took place in the first place. The goal is to use these speculative models of the prebig bang era to calculate the gravity radiation emitted by the big bang. Each of the various prebig bang theories makes different predictions. The big bang radiation predicted by the Big Splat theory, for example, differs from the radiation predicted by some of the inflation theories, so LISA might be able to rule out several of these theories. Obviously, these prebig bang models cannot be tested directly, since they involve understanding the universe before the creation of time itself, but we can test them indirectly since each of these theories predicts a different radiation spectrum emerging afterward from the big bang.
Physicist Kip Thorne writes, "Sometime between 2008 and 2030, gravitational waves from the Big Bang singularity will be discovered. There will ensue an era, lasting at least until 2050...These efforts will reveal intimate details of the Big Bang singularity, and will thereby verify that some version of string theory is the correct quantum theory of gravity."
If LISA is unable to differentiate between different prebig bang theories, its successor, the Big Bang Observer (BBO) might. It is tentatively scheduled for launch in 2025. The BBO will be able to scan the entire universe for all binary systems involving neutron stars and black holes with ma.s.s less than one thousand times the ma.s.s of the sun. But its main goal is to a.n.a.lyze gravity waves emitted during the inflationary phase of the big bang. In this sense, the BBO is specifically designed to probe the predictions of the inflationary big bang theory.
The BBO is somewhat similar to LISA in design. It will consist of three satellites moving together in an orbit around the sun, separated from each other by 50,000 kilometers (these satellites will be much closer to one another than LISA's satellites). Each satellite will be able to fire a 300-watt laser beam. BBO will be able to probe gravity wave frequencies between LIGO and LISA, filling an important gap. (LISA can detect gravity waves from 10 to 3,000 hertz, while LIGO can detect gravity waves of frequency 10 microhertz to 10 millihertz. BBO will be able to detect frequencies that include both ranges.) "By 2040 we will have used those laws [of quantum gravity] to produce high-confidence answers to many deep and puzzling questions," Thorne writes, "including...What came before the Big Bang singularity, or was there even such a thing as a 'before'? Are there other universes? And if so, how are they related to or connected to our own universe?...Do the laws of physics permit highly advanced civilizations to create and maintain wormholes for interstellar travel, and to create time machines for backward time travel?"
The point is that in the next few decades there should be enough data pouring in from gravity wave detectors in s.p.a.ce to differentiate between the various prebig bang theories.
THE END OF THE UNIVERSE.
The poet T. S. Eliot asked the question, Will the universe die with a bang or a whimper? Robert Frost asked, Will we all perish in fire or ice? The latest evidence points to the universe dying in a Big Freeze, in which temperatures will reach near absolute zero and all intelligent life will be extinguished. But can we be sure?
Some have raised another "impossible" question. How will we ever know the ultimate fate of the universe, they ask, since this event is trillions upon trillions of years in the future? Scientists believe that "dark energy" or the energy of the vacuum seems to be pus.h.i.+ng the galaxies apart at an ever increasing rate, indicating that the universe seems to be in a runaway mode. Such an expansion would cool the temperature of the universe and ultimately lead to the Big Freeze. But is this expansion temporary? Could it reverse itself in the future?
For example, in the Big Splat scenario, in which two membranes collide and create the universe, it appears as if the membranes can collide periodically. If so, then the expansion that appears to lead to a Big Freeze is only a temporary state that will reverse itself.
What is driving the current acceleration of the universes is dark energy, which in turn is probably caused by the "cosmological constant." The key, therefore, is to understand this mysterious constant, or the energy of the vacuum. Does the constant vary with time, or is it really a constant? At present, no one knows for sure. We know from the WMAP satellite currently orbiting the Earth that this cosmological constant seems to be driving the current acceleration of the universe, but we don't know if it is permanent or not.
This problem is actually an old one, dating back to 1916 when Einstein first introduced the cosmological constant. Soon after proposing general relativity the previous year, he worked out the cosmological implications of his own theory. Much to his surprise, he found that the universe was dynamic, that it either expanded or contracted. But this idea seemed to contradict the data.
Einstein was encountering the Bentley paradox, which had bedeviled even Newton. Back in 1692 the Reverend Richard Bentley wrote Newton an innocent letter with a devastating question. If Newton's gravity was always attractive, Bentley asked, then why doesn't the universe collapse? If the universe consists of a finite collection of stars that mutually attract each other, then the stars should come together and the universe should collapse into a fireball! Newton was deeply troubled by this letter, since it pointed out a key flaw in his theory of gravity: any theory of gravity that is attractive is inherently unstable. Any finite collection of stars will inevitably collapse under gravity.
Newton wrote back that the only way to create a stable universe was to have an infinite and uniform collection of stars, with each star being pulled in all directions, so that all the forces cancel out. It was a clever solution, but Newton was smart enough to realize that such stability was deceptive. Like a house of cards, the tiniest of vibrations would cause the whole thing to collapse. It was "metastable" that is, it was temporarily stable until the slightest perturbations caused it to crash. Newton concluded that G.o.d was necessary to periodically nudge the stars a bit so the universe did not collapse.
In other words, Newton saw the universe as a gigantic clock, wound up by G.o.d at the beginning of time and obeying Newton's laws. It has been ticking automatically ever since, without divine intervention. However, according to Newton, G.o.d was necessary to tweak the stars once in a while so the universe did not collapse into a fireball.
When Einstein stumbled on the Bentley paradox in 1916, his equations correctly told him that the universe was dynamic-either expanding or contracting-and that a static universe was unstable and would collapse due to gravity. But the astronomers insisted at that time that the universe was static and unchanging. So Einstein, bowing to the observations of the astronomers, added the cosmological constant, an antigravity force that pushed the stars apart to balance the gravitational pull causing the universe to collapse. (This antigravity force corresponded to the energy contained within the vacuum. In this picture even the vast emptiness of s.p.a.ce contains large quant.i.ties of invisible energy.) This constant would have to be chosen very precisely in order to cancel out the attractive force of gravity.
Later, when Edwin Hubble showed in 1929 that the universe was, in fact, expanding, Einstein would say that the cosmological constant was his "greatest blunder." Yet now, seventy years later, it appears as if Einstein's "blunder," the cosmological constant, could in fact be the largest source of energy in the universe, making up 73 percent of the matter-energy content of the universe. (By contrast, the higher elements that make up our bodies const.i.tute only .03 percent of the universe.) Einstein's blunder will likely determine the ultimate fate of the universe.
But where does this cosmological constant come from? At present no one knows. At the beginning of time, the antigravity force was perhaps large enough to cause the universe to inflate, creating the big bang. Then it suddenly disappeared, for reasons that are unknown. (The universe was still expanding during this period, but at a slower pace.) And then, about eight billion years after the big bang, the antigravity force resurfaced again, causing the galaxies to push out and causing the universe to accelerate once again.
So is it "impossible" to determine the ultimate fate of the universe? Perhaps not. Most physicists believe that quantum effects ultimately determine the size of the cosmological constant. A naive calculation, using a primitive version of the quantum theory, shows that the cosmological constant is off by a factor of 10120. This is the greatest mismatch in the history of science.
But there is also a consensus among physicists that this anomaly simply means that we need a theory of quantum gravity. Since the cosmological constant arises via quantum corrections, it is necessary to have a theory of everything-a theory that will allow us to calculate not just the Standard Model, but also the value of the cosmological constant, which will determine the ultimate fate of the universe.
So a theory of everything is necessary to determine the ultimate fate of the universe. The irony is that some physicists believe that it is impossible to attain a theory of everything.
A THEORY OF EVERYTHING?.
As I mentioned earlier, string theory is the leading candidate for a "theory of everything," yet there are opposing camps on whether the string theory lives up to this claim. On the one hand, people like MIT professor Max Tegmark write, "In 2056, I think you'll be able to buy a T-s.h.i.+rt on which are printed equations describing the unified physical laws of our universe." On the other hand, there is an emerging band of determined critics who claim that the string bandwagon has yet to deliver. No matter how many breathless articles or TV doc.u.mentaries are produced concerning string theory, it has yet to produce a single testable fact, some say. It's a theory of nothing, rather than a theory of everything, claim the critics. The debate heated up considerably in 2002 when Stephen Hawking switched sides, quoting the incompleteness theorem, and said that a theory of everything might even be mathematically impossible.
It's not surprising that the debate has pitted physicist against physicist, because the goal is so lofty, if elusive. The quest to unify all the laws of nature has tantalized and lured philosophers and physicists alike for millennia. Socrates himself once said, "It seemed to me a superlative thing-to know the explanation of everything, why it comes to be, why it perishes, why it is."
The first serious proposal for a theory of everything dates back to about 500 BC, when the Greek Pythagoreans are credited with deciphering the mathematical laws of music. By a.n.a.lyzing the nodes and vibrations of a lyre string, they showed that music obeyed remarkably simple mathematics. They then speculated that all of nature could be explained in the harmonies of the lyre string. (In some sense, string theory brings back the dream of the Pythagoreans.) In modern times nearly all the giants of twentieth-century physics tried their luck with a unified field theory. But, as Freeman Dyson cautions, "The ground of physics is littered with the corpses of unified theories."
In 1928 the New York Times ran the sensational headline "Einstein on verge of great discovery; resents intrusion." The news story helped spark a media feeding frenzy over a theory of everything that was whipped up to a feverish pitch. Headlines blared "Einstein is amazed at stir over theory. Holds 100 journalists at bay for a week." Scores of journalists swarmed around his home in Berlin, maintaining a non-stop vigil, waiting to catch a glimpse of the genius and grab a headline. Einstein was forced to go into hiding.
Astronomer Arthur Eddington wrote to Einstein: "You may be amused to hear that one of our great department stores in London (Selfridges) has posted on its window your paper (the six pages pasted up side by side) so that pa.s.sers-by can read it all through. Large crowds gather around to read it." (In 1923 Eddington proposed his own unified field theory on which he worked tirelessly for the rest of his life, until he died in 1944.) In 1946 Erwin Schrodinger, one of the founders of quantum mechanics, held a press conference to propose his unified field theory. Even Ireland's Prime Minister, Eamon De Valera, showed up. When a reporter asked him what he would do if his theory was wrong, Schrodinger replied, "I believe I am right. I shall look like an awful fool if I am wrong." (Schrodinger was humiliated when Einstein politely pointed out the errors in his theory.) The harshest of all critics of unification was physicist Wolfgang Pauli. He chided Einstein, saying, "What G.o.d has torn asunder, let no man put together." He mercilessly put down any half-baked theory with the quip: "It's not even wrong." So it is ironic that the supreme cynic Pauli himself caught the bug. In the 1950s he proposed his own unified field theory with Werner Heisenberg.
In 1958 Pauli presented the Heisenberg-Pauli unified theory at Columbia University. Niels Bohr was in the audience, and he was not impressed. Bohr stood up and said, "We in the back are convinced that your theory is crazy. But what divides us is whether your theory is crazy enough." The criticism was crus.h.i.+ng. Since all the obvious theories had been considered and rejected, the true unified field theory must be a dazzling departure from the past. The Heisenberg-Pauli theory was simply too conventional, too ordinary, too sane to be the true theory. (That year Pauli was disturbed when Heisenberg commented on a radio broadcast that only a few technical details were left in their theory. Pauli sent his friends a letter with a blank rectangle, with the caption, "This is to show the world I can paint like t.i.tian. Only technical details are missing.") CRITICISMS OF STRING THEORY.
Today the leading (and only) candidate for a theory of everything is string theory. But, again, a backlash has arisen. Opponents claim that to get a tenured position at a top university you have to work on string theory. If you don't you will be unemployed. It's the fad of the moment, and it's not good for physics.
I smile when I hear this criticism, because physics, like all human endeavors, is subject to fads and fas.h.i.+ons. The fortunes of great theories, especially on the cutting edge of human knowledge, can rise and fall like hemlines. In fact, years ago the tables were turned; string theory was historically an outcast, a renegade theory, the victim of the bandwagon effect.
String theory was born in 1968, when two young postdocs, Gabriel Veneziano and Mahiko Suzuki, stumbled on a formula that seemed to describe the collisions of subatomic particles. Quickly it was discovered that this marvelous formula could be derived by the collision of vibrating strings. But by 1974 the theory was dead in its tracks. A new theory, quantum chromodynamics (QCD), or the theory of quarks and the strong interaction, was a juggernaut flattening all other theories. People left string theory in droves to work on QCD. All the funding, jobs, and recognition went to physicists working on the quark model.
I remember those dark years well. Only the foolhardy or the stubborn persisted in working on string theory. And when it became known that these strings could vibrate only in ten dimensions, the theory became the b.u.t.t of jokes. String pioneer John Schwarz at Cal Tech would sometimes b.u.mp into Richard Feynman in the elevator. Ever the joker, Feynman would ask, "Well, John, and how many dimensions are you in today?" We used to joke that the only place to find a string theorist was in the unemployment line. (n.o.bel laureate Murray Gell-Mann, founder of the quark model, once confided to me that he took pity on string theorists and created a "nature preserve for endangered string theorists" at Cal Tech so people like John wouldn't lose their jobs.) Given that today so many young physicists are rus.h.i.+ng to work on string theory, Steve Weinberg has written, "String theory provides our only present source of candidates for a final theory-how could anyone expect that many of the brightest young theorists would not work on it?"
IS STRING THEORY UNTESTABLE?.
One major criticism of string theory today is that it is untestable. It would take an atom smasher the size of the galaxy to test this theory, critics claim.
But this criticism neglects the fact that most science is done indirectly, not directly. No one has ever visited the sun to do a direct test, but we know it is made of hydrogen because we can a.n.a.lyze its spectral lines.
Or take black holes. The theory of black holes dates back to 1783, when John Mich.e.l.l published an article in the Philosophical Transactions of the Royal Society. He claimed that a star could be so ma.s.sive that "all light emitted from such a body would be made to return to it by its own proper gravity." Mich.e.l.l's "dark star" theory languished for centuries because a direct test was impossible. In 1939 Einstein even wrote a paper showing that such a dark star could not form by natural means. The criticism was that these dark stars were inherently untestable because they were, by definition, invisible. Yet today the Hubble s.p.a.ce Telescope has given us gorgeous evidence of black holes. We now believe that billions of them could lurk in the hearts of galaxies; scores of wandering black holes could exist in our own galaxy. But the point is that the evidence for black holes is all indirect; that is, we have gathered information about black holes by a.n.a.lyzing the accretion disk that swirls around them.
Furthermore, many "untestable" theories ultimately become testable. It took two thousand years to prove the existence of atoms after they were first proposed by Democritus. Nineteenth-century physicists such as Ludwig Boltzmann were hounded to death for believing in that theory, yet today we have gorgeous photographs of atoms. Pauli himself introduced the concept of the neutrino in 1930, a particle so elusive it can pa.s.s through blocks of solid lead the size of an entire star system and not be absorbed. Pauli said, "I have committed the ultimate sin; I have introduced a particle that can never be observed." It was "impossible" to detect the neutrino, so it was considered little more than science fiction for several decades. Yet today we can produce beams of neutrinos.
There are, in fact, a number of experiments that will provide, physicists hope, the first indirect tests of string theory: The Large Hadron Collider (LHC) might be powerful enough to produce "sparticles," or superparticles, which are the higher vibrations predicted by superstring theory (as well as by other supersymmetric theories).
As I mentioned earlier, in 2015 the Laser Interferometer s.p.a.ce Antenna (LISA) will be launched in s.p.a.ce. LISA and its successor, the Big Bang Observer, may be sensitive enough to test several "prebig bang" theories, including versions of the string theory.
A number of labs are investigating the presence of higher dimensions by looking at deviations from Newton's famed inverse-square law at the millimeter scale. (If there is a fourth spatial dimension, then gravity should fall by the inverse cube, not the inverse square.) The latest version of string theory (M-theory) predicts there are eleven dimensions.
Many labs are trying to detect dark matter, since the Earth is moving in a cosmic wind of dark matter. String theory makes specific, testable predictions about the physical properties of dark matter because dark matter is probably a higher vibration of the string (e.g., the photino).
It is hoped that a series of additional experiments (e.g., on neutrino polarization in the south pole) will detect the presence of miniblack holes and other strange objects by a.n.a.lyzing anomalies in cosmic rays, whose energies can easily exceed those of the LHC. Cosmic ray experiments and the LHC will open a new, exciting frontier beyond the Standard Model.
And there are some physicists who hold out the possibility that the big bang was so explosive that perhaps a tiny superstring was blown up into astronomical proportions. As physicist Alexander Vilenkin of Tufts University writes, "A very exciting possibility is that superstrings...can have astronomical dimensions...We would then be able to observe them in the sky and directly test superstring theory." (The probability of finding a huge, relic superstring that was blown up during the big bang is quite small.) IS PHYSICS INCOMPLETE?.
In 1980 Stephen Hawking helped to spark interest in a theory of everything with his lecture ent.i.tled "Is the End in Sight for Theoretical Physics?" in which he said, "We may see a complete theory within the lifetime of some of those present here." He claimed that there was a fifty-fifty chance that the final theory would be found in the next twenty years. But when the year 2000 arrived and there was no consensus on the theory of everything, he changed his mind and said that there was a fifty-fifty chance of finding it in another twenty years.
Then in 2002 Hawking changed his mind once again, declaring that G.o.del's incompleteness theorem may suggest a fatal flaw in his original line of thinking. He wrote, "Some people will be very disappointed if there is not an ultimate theory that can be formulated as a finite number of principles. I used to belong to that camp, but I have changed my mind...G.o.del's theorem ensured there would always be a job for mathematicians. I think M-theory will do the same for physicists."
His argument is an old one: since mathematics is incomplete and the language of physics is mathematics, there will always be true physical statements that are forever beyond our reach, and hence a theory of everything is not possible. Since the incompleteness theorem killed off the Greek dream of proving all true statements in mathematics, it will also put a theory of everything forever beyond our reach.
Freeman Dyson said it eloquently when he wrote, "G.o.del proved the world of pure mathematics is inexhaustible; no finite set of axioms and rules of inference can ever encompa.s.s the whole of mathematics...I hope that an a.n.a.logous situation exists in the physical world. If my view of the future is correct, it means that the world of physics and astronomy is also inexhaustible; no matter how far we go into the future, there will always be new things happening, new information coming in, new worlds to explore, a constantly expanding domain of life, consciousness, and memory."
Astrophysicist John Barrow summarizes this logic this way: "Science is based on mathematics; mathematics cannot discover all truths; therefore science cannot discover all truths."
Physics of the Impossible Part 12
You're reading novel Physics of the Impossible Part 12 online at LightNovelFree.com. You can use the follow function to bookmark your favorite novel ( Only for registered users ). If you find any errors ( broken links, can't load photos, etc.. ), Please let us know so we can fix it as soon as possible. And when you start a conversation or debate about a certain topic with other people, please do not offend them just because you don't like their opinions.
Physics of the Impossible Part 12 summary
You're reading Physics of the Impossible Part 12. This novel has been translated by Updating. Author: Michio Kaku already has 1299 views.
It's great if you read and follow any novel on our website. We promise you that we'll bring you the latest, hottest novel everyday and FREE.
LightNovelFree.com is a most smartest website for reading novel online, it can automatic resize images to fit your pc screen, even on your mobile. Experience now by using your smartphone and access to LightNovelFree.com
- Related chapter:
- Physics of the Impossible Part 11
- Physics of the Impossible Part 13
RECENTLY UPDATED NOVEL

The Strongest War God
The Strongest War God Chapter 1302: A Filial Son and a Kind Father View : 454,556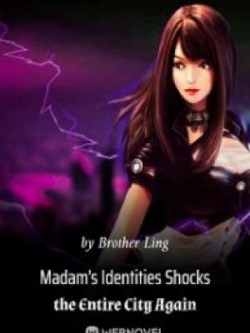
Madam's Identities Shocks The Entire City Again
Madam's Identities Shocks The Entire City Again Chapter 3417: Where's Your Patriarch? I Want to See Him! View : 2,640,772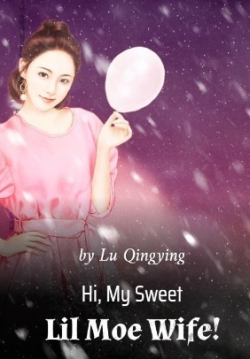
Hi, My Sweet Lil Moe Wife!
Hi, My Sweet Lil Moe Wife! Chapter 2312: What kind of godly brother is this? View : 482,852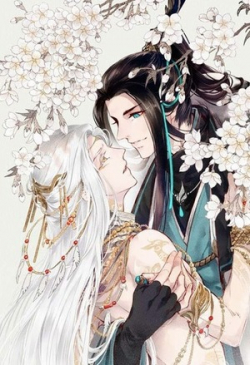