Concrete Construction Part 9
You’re reading novel Concrete Construction Part 9 online at LightNovelFree.com. Please use the follow button to get notification about the latest chapter next time when you visit LightNovelFree.com. Use F11 button to read novel in full-screen(PC only). Drop by anytime you want to read free – fast – latest novel. It’s great if you could leave a comment, share your opinion about the new chapters, new novel with others on the internet. We’ll do our best to bring you the finest, latest novel everyday. Enjoy!
[Ill.u.s.tration: Fig. 32.--Form for Molding Footing for Block Concrete Breakwater.]
The bag shown by Fig. 31 was used to deposit concrete for leveling up a rough rock bottom and so provide a footing for a concrete block pier constructed in 1902 at Peterhead, N. B., by Mr. William s.h.i.+eld, M. Inst.
C. E. Careful longitudinal profiles were taken of the rock bottom one at each edge of the footing. Side forms were then made in 20-ft. sections as shown by Fig. 32; the lagging boards being cut to fit the determined profile and the top of the longitudinal piece being flush with the top of the proposed footing. The concrete was filled in between the side forms and leveled off by the T-rail straight-edge. In placing the side forms the longitudinal pieces were placed by divers who were given the proper elevations by level rods having 10 to 15-ft. extension pieces to raise the targets above the water surface. When leveled the side pieces were anchor-bolted as shown to the rock, the anchor-bolts being wedged into the holes to permit future removal. The concrete was then lowered in the bag shown by Fig. 31, the divers a.s.sisting in guiding the bag to position. The mouth of the bag being tied by one turn of a line having loops through which a wooden key is slipped to hold the line tight, a sharp tug on the tripping rope loosens the key and empties the bag. The bags used on this work had a capacity of 2 cu. ft. To permit the removal of the side forms after the concrete had hardened, a strip of jute sacking was spread against the lagging boards with a flap extending 15 to 18 ins. under the concrete. The forms were removed by divers who loosened the anchor bolt wedges.
In placing small amounts of concrete for bridge foundations in Nova Scotia, bags, made of rough brown paper were used to hold the concrete.
Each bag held about 1 cu. ft. The bags were made up quickly and dropped into the water one after the other so that the following one was deposited before the cement escaped from the former one. The paper was immediately destroyed by submersion and concrete remained. The bags cost $1.35 per hundred or 35 cts. per cu. yd. of concrete. Concrete was thus deposited in 18 ft. of water without a diver.
[Ill.u.s.tration: Fig. 33.--Steel Tremie for Depositing Concrete Under Water.]
~DEPOSITING THROUGH A TREMIE.~--A tremie consists of a tube of wood or, better, of sheet metal, which reaches from above the surface to the bottom of the water; it is operated by filling the tube with concrete and keeping it full by successive additions while allowing the concrete to flow out gradually at the bottom by raising the tube slightly to provide the necessary opening. A good example of a sheet steel tremie is shown by Fig. 33. This tremie was used by Mr. Wm. H. Ward in constructing the Harvard Bridge foundations and numerous other subaqueous structures of concrete. In these works the tube was suspended from a derrick. Wheelbarrows filled the tube and hopper with concrete and kept them full; the derrick raised the tube a few inches and swung it gently so as to move it slowly over the area to be filled. Care being taken to keep the tube at one height, the concrete was readily deposited in even layers. Concrete thus deposited in 18 ft. of water was found to be level and solid on pumping the pit dry.
[Ill.u.s.tration: Fig. 34.--Tremie and Traveler Used at Charlestown, Ma.s.s., Bridge.]
Another method of handling a tremie was employed in constructing the foundations for the Charlestown Bridge at Boston, Ma.s.s. Foundation piles were driven and sawed off under water. A frame was built above water and supported by a curbing attached to certain piles in the outer rows of the foundation reserved for this purpose. In this frame the vertical members were Wakefield sheet-piling plank, s.p.a.ced 6 to 10 ft. apart, and connected by three lines of double waling bolted to the verticals at three different heights. This frame was lowered to the bottom so as to enclose the bearing piles. The posts or verticals were then driven, one by one, into the bottom, the frame being flexible enough to permit this.
The s.p.a.ces between the posts or verticals were then filled by sheet-piling and the frame was bolted to the curbing piles. This curbing afterward supported the traveler used in laying the concrete. Thus a coffer dam was formed to receive the concrete as shown in Fig. 34. The 1-2-5 concrete was deposited up to within 5 ft. of the mean low water level, the last foot being laid after water was pumped out. The tremie used to deposit the concrete was a tube 14 ins. in diameter at the bottom and 11 ins. at the neck, with a hopper at the top. It was made in removable sections, with outside f.l.a.n.g.es, and was suspended by a differential hoist from a truck moving laterally on a traveler, Fig. 34.
The foot of the chute rested on the bottom until filled with concrete; then the chute was slowly raised and the concrete allowed to run but into a conical heap, more concrete being dumped into the hopper. As the truck moved across the traveler a ridge of concrete was made; then the traveler was moved forward and another parallel ridge was made. The best results were obtained when the layers were 2 ft. thick, but layers up to 6 ft. thick were laid. If the layer was too thick, or uneven, or if the chute was moved or raised too quickly, the charge in the tube was "lost." This was objectionable because the charging of the chute anew resulted in "was.h.i.+ng" the cement more or less out of the concrete until the chute was again filled. To reduce this objection the contractor was directed to dump some neat cement into the tube before filling with concrete. A canva.s.s piston was devised which could be pushed ahead of the concrete when filling the chute. It consisted of two truncated cones of canva.s.s, one flaring downward to force the water ahead, and the other flaring upward to hold the concrete. The canva.s.s was stiffened and held against the sides of the chute by longitudinal ribs of spring steel wire; the waist was filled by a thick block of wood to which all the springs were attached; and to this block were connected additional steel guides to prevent overturning and a rope to regulate the descent. Very little water forced its way past this piston and it was a success, but as the cost was considerable and a piston was lost each time, its use was abandoned as the evil to be avoided did not justify the outlay.
The chute worked best when the concrete was mixed not quite wet enough to be plastic. If mixed too wet the charge was liable to be "lost," and if dry it would choke the chute. An excess of gravel permitted water to ascend in the tube; and an excess of sand tended to check the flow of concrete.
In constructing the piers for a masonry arch bridge in France in 1888 much the same method was followed, except that a wooden tremie 16 ins.
square made in detachable sections was used. This tremie had a hopper top and was also provided with a removable cap or cover for the bottom end, the latter device being intended to keep the water out of the tube and prevent "was.h.i.+ng" the first charge of concrete. The piers were constructed by first driving piles and sawing them off several feet above the bottom but below water level, and then filling them nearly to their tops with broken stone. An open box caisson was then sunk onto the stone and embracing the pile tops and then filled around the outside with more broken stone. The caisson was then filled with concrete through the tremie which was handled by a traveling crane. The crane was mounted and traveled transversely of the pier on a platform which in turn moved along tracks laid lengthwise of the caisson. The tube was gradually filled with concrete and lowered, the detachable bottom of the tube was then removed, allowing the concrete to run out. The tube was first moved across the caisson and then downstream and back across the caisson, and this operation repeated until a 16-in. layer was completed.
The tube was then raised 16 ins. and the operations repeated to form another layer. There was almost no _laitance_. From 90 to 100 cu. yds.
were deposited daily.
Still another example of tremie work is furnished by the task of depositing a large ma.s.s of concrete under water in the construction of the Nussdorf Lock at Vienna. This lock has a total width of 92 ft. over all, and is 49.2 ft. clear inside. The excavation, which was carried to a depth of 26.24 ft. below water level, was made full width, between sheet piling, and the bottom was filled in with rammed sand and gravel, forming a kind of invert with its upper surface horizontal in the middle and sloping upwards a trifle at both sides. A ma.s.s of concrete having a total thickness of 13.12 ft. was built on this foundation in the center where the upper surfaces were 13.12 ft. below the water level. Concrete walls were carried up at the sides of the lock to a height of 3.28 ft.; these walls were 8.2 ft. thick. The methods used in placing the concrete were as follows: Three longitudinal rows of piles were driven on each side of the axis of the lock, these piles supporting a 6-rail track about 7 ft. above the water level. Three carriages spanning the full width of the lock transversely moved on this track. Each carriage had three trolleys, one in each of the main panels of the transverse pile bends. These trolleys each carried a vertical telescopic tube, by means of which the concrete was deposited at the bottom of the lock. These tubes or chutes were of different lengths in the three carriages; the first ones deposited the concrete up to a level of 23 ft. below the surface; the next set deposited the concrete between that level and 19.7 ft., and the last set completed the subaqueous work up to the final height of 16.4 ft. below the surface. The tops of the tubes were level with a transverse track extending the full length of the carriage. The ends of these tracks just cleared the outside rows of piles, which, on one side of the lock, supported a distribution track parallel to the axis of the lock. Dump cars running on this distribution track delivered the concrete to smaller dump cars on the carriage tracks, and in turn these smaller cars dumped into either of these chutes on each carriage.
The carriages were moved from end to end of the lock, the whole area of the lock coming under the nine chutes, inasmuch as each chute moved one-third the length of the carriage. The concrete was deposited in three horizontal layers 3.28 ft. thick, the layers being built in comparatively narrow banks, so that the different layers would key together and form a corrugated ma.s.s. The chutes were shortened as the concrete was deposited, three layers being placed successively. The main body of the bottom and the side walls were built by this method, and then the water was pumped out and a 2.3 ft. layer of concrete rammed over the bottom and completed with a finished surface 9 ft. thick.
~GROUTING SUBMERGED STONE.~--Ma.s.ses of gravel, broken or rubble stone deposited under water may be cemented into virtually a solid concrete by charging the interstices with grout forced through pipes from the surface. Mr. H. F. White gives the following records of grouting submerged gravel:
In experiment No. 1 a reservoir 10 ft. square was filled to a depth of 18 ins. with clean gravel ballast (1 to 2-in. size) submerged in water.
A 2-in. gas pipe rested on the gravel and was surmounted with a funnel.
A 1:1 Portland grout was poured in. After 21 days set the water was drawn off, and it was found that the grout had permeated the ballast for a s.p.a.ce of 8 ft. square at the bottom and 6 ft. square at the top, leaving a small pile of pure cement mortar 6 ins. high about the base of the pipe; 16 cu. ft. of cement and 16 cu. ft. of sand concreted 100 cu.
yds. of ballast. In experiment No. 2, under the same conditions, a grout made of 1 part lime, 1 part surki (puzzulana or tra.s.s) and 1 part sand, was found to have spread over the entire bottom, 10 ft. square, rising 5 ins. on the sides, and making the concreted ma.s.s about 3 ft. square at the top; 25 cu. ft. of the dry materials concreted 100 cu. ft. of ballast. In experiment No. 3 the ballast was 2 ft. deep. A grout (using 8 cu. ft. of each ingredient) made as in experiment No. 2 covered the bottom, rose 14 ins. on the sides and made a top surface 4 ft. square; 32 cu. ft. of the dry materials grouted 100 cu. ft. of ballast. In experiment No. 4 the ballast was of bats and pieces 3 or 4 ins. in size laid 7 ft. deep. A grout made as in experiment No. 2 (using 88 cu. ft.
of each ingredient) concreted the whole ma.s.s to a depth of 6 ft. up the sides, and 2 ft. square at the pipe on the surface of the ballast. Mr.
White says that a grout containing more than 1 part of sand to 1 of Portland cement will not run freely through a 2-in. pipe, as the sand settles out and chokes the pipe. Even with 1:1 grout it must be constantly stirred and a steady flow into the pipe maintained. The lime-tra.s.s grout does not give the same trouble.
Mr. W. R. Knipple describes the work of grouting rubble stone and gravel for the base of the Hermitage Breakwater. This breakwater is 525 ft.
long, 50 ft. wide at base and 42 ft. wide at top, and 68 ft. high, was built on the island of Jersey. Where earth (from 0 to 8 ft. deep) overlaid the granite rock, it was dredged and the trench filled in with rubble stones and gravel until a level foundation was secured. Cement grout was then forced into this filling through pipe placed 8 to 10 ft.
apart. The grouting was done in sections 12 ft. long, from 7 to 10 days being taken to complete each. Upon this foundation concrete blocks, 449 to 12 ft., were laid in courses inclined at an angle of 68. The first four courses were laid by divers, the blocks being stacked dry two courses high at a time. The joints below water were calked by divers and above water by masons, and a section was then grouted. When two courses had been laid and grouted, two more courses were laid and grouted in turn, and so on. In places, grouting was done in 50 ft. of water. The grout should be a thick paste; a 30-ft. column of grout will balance a 60-ft. column of water.
CHAPTER VI.
METHODS AND COST OF MAKING AND USING RUBBLE AND ASPHALTIC CONCRETE.
Two kinds of concrete which vary in composition and character from the common standard mixtures of cement, sand and broken aggregate are extensively employed in engineering construction. These are rubble concrete and asphaltic concrete.
~RUBBLE CONCRETE.~--In constructing ma.s.sive walls and slabs a reduction in cost may often (not always) be obtained by introducing large stones into the concrete. Concrete of this character is called rubble concrete, and the percentage of rubble stone contained varies from a few per cent. to, in some cases, over half of the volume. The saving effected comes partly from the reduction in the cement required per cubic yard of concrete and partly from the saving in crus.h.i.+ng.
The saving in cement may be readily figured if the composition of the concrete and the volume of the added rubble stones be known. A 1-2-5 concrete requires according to Table X in Chapter II 1.13 bbls. of cement per cubic yard. a.s.suming a barrel of cement to make 3.65 cu. ft.
of paste, we have 3.65 1.13 = 4.12 cu. ft. of cement paste per cubic yard of 1-2-5 concrete. This means that about 15 per cent. of the volume of the concrete structure is cement. If rubble stone be introduced to 50 per cent. of the volume, then the structure has about 7 per cent. of its volume of cement. It is of interest to note in this connection that rubble masonry composed of 65 per cent. stone and 35 per cent. of 1-2 mortar would have some 11 per cent. of its volume made up of cement.
The saving in crus.h.i.+ng is not so simple a determination. Generally speaking, the fact that a considerable volume of the concrete is composed of what, we will call uncrushed stone, means a saving in the stone const.i.tuent of one structure amounting to what it would have cost to break up and screen this volume of uncrushed stone, but there are exceptions. For example, the anchorages of the Manhattan Bridge over the East River at New York city were specified to be of rubble concrete, doubtless because the designer believed rubble concrete to be cheaper than plain concrete. In this case an economic mistake was made, for all the rubble stone used had to be quarried up the Hudson River, loaded onto and s.h.i.+pped by barges to the site and then unloaded and handled to the work using derricks. Now this repeated handling of large, irregular rubble stones is expensive. Crushed stone as we have shown in Chapter IV can be unloaded from boats at a very low cost by means of clam sh.e.l.ls.
It can be transported on a belt conveyor, elevated by bucket conveyer, mixed with sand and cement and delivered to the work all with very little manual labor when the installation of a very efficient plant is justified by the magnitude of the job. Large rubble stones cannot be handled so cheaply or with so great rapidity as crushed stone; the work may be so expensive, due to repeated handlings, as to offset the cost of crus.h.i.+ng as well as the extra cost of cement in plain concrete. On the other hand, the cost of quarrying rock suitable for rubble concrete is no greater than the cost of quarrying it for crus.h.i.+ng--it is generally less because the stone does not have to be broken so small--so that when the cost of getting the quarried rock to the crusher and the crushed stone into the concrete comes about the same as getting the quarried stone into the structure it is absurd practice to require crus.h.i.+ng. To go back then to our first thought, the question whether or not saving results from the use of rubble concrete, is a separate problem in engineering economics for each structure.
In planning rubble concrete work the form of the rubble stones as they come from the quarry deserves consideration. Stones that have flat beds like many sandstones and limestones can be laid upon layers of dry concrete and have the vertical interstices filled with dry concrete by tamping. It requires a sloppy concrete to thoroughly embed stones which break out irregularly. In the following examples of rubble concrete work the reader will find structures varying widely enough in character and in the percentages of rubble used to cover most ordinary conditions of such work.
Where the rubble stones are very large it is now customary to use the term "cyclopean masonry" instead of rubble concrete. Many engineers who have not studied the economics of the subject believe that the use of ma.s.sive blocks of stone bedded in concrete necessarily gives the cheapest form of masonry. We have already indicated conditions where ordinary concrete is cheaper than rubble concrete. We may add that if the quarry yields a rock that breaks up naturally into small sized blocks, it is the height of economic folly to specify large sized cyclopean blocks. Nevertheless this blunder has been frequently made in the recent past.
[Ill.u.s.tration: Fig. 35.--Diagram Cross-Section of Rubble Concrete Dam, Chattahoochee River.]
~Chattahoochee River Dam.~--The roll-way portion, 680 ft. long, of the dam for the Atlanta Water & Electric Power Co., shown in section by Fig. 35, was built of a hearting of rubble concrete with a fine concrete facing and a rubble rear wall. The facing, 12 ins. thick of 1-2-4 concrete, gave a smooth surface for the top and face of the dam, while the rubble rear wall enabled back forms to be dispensed with and, it was considered, made a more impervious masonry. The concrete matrix for the core was a 1-2-5 stone mixture made very wet. The rubble stones, some as large as 4 cu. yds., were bedded in the concrete by dropping them a few yards from a derrick and "working" them with bars; a well formed stone was readily settled 6 ins. into a 10-in. bed of concrete. The volume of rubble was from 33 to 45 per cent. of the total volume of the masonry.
The 1-2-4 concrete facing was brought up together with the rubble core, using face forms and templates to get the proper profile. The work was done by contract and the average was 5,500 cu. yds. of concrete placed per month.
[Ill.u.s.tration: Fig. 36.--Cross-Section of Barossa Dam of Rubble Concrete.]
~Barossa Dam, South Australia.~--The Barossa Dam for the water-works for Gawler, South Australia, is an arch with a radius of 200 ft., and an arc length on top of 422 ft.; its height above the bed of the stream is 95 ft. Figure 36 is a cross-section of the dam at the center. The dam contains 17,975 cu. yds. of rubble concrete in the proportions of 2,215 cu. yds. of rubble stone to 15,760 cu. yds. of concrete; thus about 12.3 per cent. of the dam was of rubble. The concrete was mixed by weight of 1 part cement, 1 parts sand, and a varying proportion of aggregate composed of 4 parts 1 to 2-in. stone, 2 parts to 1-in. stone and 1 part 1/8 to -in. stone or screenings. The sand was one-half river sand and one-half crusher sand. The following shows the amounts by weight of the several materials for each of the several cla.s.ses of concrete per cubic yard:
-------------Stone---------- Cla.s.s. Excess Mortar. 1-2. -1. 1/8-. Sand. Cement.
A 7.5% 1,500 661 333 804 434 B 12.5 1,433-1/3 637 318 858 463 C 12.5 1,434 637 318 859 474 D 15 1,402 623 312 884 484
[Ill.u.s.tration: Fig. 37.--Apparatus Used for Weighing Concrete Materials at Barossa Dam.]
The average composition of the concrete was 1-1-3. Its cost per cubic yard in place including rubble was 38s 9d per cu. yd. or about $9.30. In proportioning the mixture on the work use was made of the device shown by Fig. 37 to weigh the aggregate. The measuring car is pushed back under the stone hopper chute until the wheels drop into shallow notches in the balanced track rails; stone is then admitted until the lead weight begins to rise, when the car is pushed forward and dumps automatically as indicated.
~Other Rubble Concrete Dams.~--Rubble concrete containing from 55 to 60 per cent. rubble was used in constructing the Boonton Dam at Boonton, N.
J. The stones used measured from 1 to 2 cu. yds. each; the concrete was made so wet that when the stones were dropped into it, it flowed into every crevice. The materials were all delivered on cars, from which they were delivered to the dam by derricks provided with bull-wheels. On the dam there were 4 laborers and 1 mason to each derrick, and this gang dumped the concrete and joggled the rubble stones into it. Records of 125 cu. yds. per 10 hours, with one derrick, were made. With 35 derricks, 20 of which were laying masonry and 15 either pa.s.sing materials or being moved, as much as 21,000 cu. yds. of masonry were laid in one month. The amount of cement per cubic yard of masonry is variously stated to have been 0.6 to 0.75 bbl. The stone was granite.
The Spier Falls Dam on the upper Hudson River was built of rubble concrete containing about 33 per cent. rubble stone. The concrete was a 1-2-5 mixture, and the engineer states that about 1 bbl. of cement was used per cubic yard of rubble concrete. This high percentage of cement may be accounted for by the fact that there was a considerable amount of rubble masonry in cement mortar included in the total. The stones and concrete were delivered along the dam by cableways and stiff-leg derricks set on the downstream sloping face of the dam delivered them from the cableways into place. There were two laborers to each mason employed in placing the materials, wages being 15 and 35 cts. per hour, respectively. The labor cost of placing the materials was 60 cts. per cubic yard of masonry. The stone was granite.
Granite rubble laid in layers on beds of concrete and filled between with concrete was used in constructing the Hemet Dam in California. The concrete was a 1-3-6 mixture, and was thoroughly tamped under and between the stones. For face work the stones were roughly scabbled to shape and laid in mortar. The stone was taken from the quarry 400 ft.
away and delivered directly on the dam by cableways; here two derricks handled the stone into place, the dam being only 246 ft. arc length on top, though it was 122 ft. high. The cableways would take a 10-ton load; stones could be taken from the quarry, hoisted 150 ft. and delivered to the work in 40 to 60 seconds. Common labor at $1.75 per day was used for all masonry except facing, where masons at $3.50 were employed. Cement cost delivered $5 per barrel, of which from $1 to $1.50 per barrel was the cost of hauling 23 miles by team over roads having 18 per cent. grades in places. Sand was taken from the stream bed and delivered to the work by bucket conveyor. "Under favorable conditions some of the masonry was put in for as low as $4 per cu. yd." There were 31,100 cu. yds. of masonry in the dam, which required 20,000 bbls. of cement, or 0.64 bbl. per cubic yard.
The following novel method of making rubble concrete was employed in enlarging two old dams and in constructing two new dams for a small water-works. The available time was short, the amount of work was too small and too scattered to justify the installation of a stone crusher, and suitable gravel was not at hand. Sufficient small boulders in old walls, and borrow pits and on surface of fields were available, and were used with thin Portland cement mortar. One part of Alpha or Lehigh cement and three parts sand were mixed dry at first and then wet with just enough water to make the resulting mortar flow by gravity. This mortar was shoveled into the forms continuously by one set of men while other men were throwing into the mortar in the forms the boulders which were cleaned and broken so as not to be more than 7 ins. long. In general the performance was continuous. Three mortar beds were placed parallel with, and against, one side of the forms, with s.p.a.ces of about 4 ft. between the ends of the beds. The boulders were dumped on the opposite side of the forms. Two men shoveled in all the mortar and did nothing else. While they were emptying one bed the mortar was being mixed in the preceding bed by two other men and the materials placed in the third bed by still others. Another gang was continually throwing in the boulders and small stones and still another was breaking stone. One man should keep the mortar well stirred while the bed is being emptied.
About 20 men were necessary to do all parts of the work. The forms were of 2-in. planed plank tongued and grooved. Especial pains were taken to make the forms tight, and all leaks that appeared were quickly stopped with dry cement. Some pains were taken to prevent a flat side of large stones from coming in direct contact with the forms, but round boulders and small stones needed no care to prevent their showing in the finished work.
[Ill.u.s.tration: Fig. 38.--Bridge Abutment of Rubble Concrete.]
In conclusion it is interesting to note, perhaps, the earliest use of rubble concrete for dam construction in this country in constructing the Boyd's Corner Dam on the Croton River near New York. This dam was begun in 1867 and for a time rubble concrete was used, but was finally discontinued, due to the impression that it might not be watertight. The specifications called for dry concrete to be thoroughly rammed in between the rubble stones, and to give room for this ramming the contractor was not permitted to lay any two stones closer together than 12 ins. As a result not more than 33 per cent. of the concrete was rubble.
~Abutment for Railway Bridge.~--Figure 38 shows a bridge abutment built of rubble concrete at a cost of about $4.50 per cu. yd. The concrete was a 1-2-4 mixture laid in 4-in. layers. On each layer were laid large rubble stones bedded flat and s.p.a.ced to give 6-in. vertical joints; the vertical joints were filled with concrete by ramming and then another layer of concrete placed and so on. A force of 28 men and a foreman averaged 40 cu. yds. of rubble concrete per day. The following is the itemized cost per cubic yard, not including forms, for 278 cu. yds:
Item. Per Cu. Yd.
0.82 bbls. cement, at $2.60 $2.14 0.22 cu. yd. sand, at $1.00 0.22 0.52 cu. yd. broken stone, at $0.94 0.49 0.38 cu. yd. rubble stone, at $0.63 0.24 Water 0.07 Labor, at 15 cts. per hour 1.19 Foreman 0.09 ------- Total $4.44
Concrete Construction Part 9
You're reading novel Concrete Construction Part 9 online at LightNovelFree.com. You can use the follow function to bookmark your favorite novel ( Only for registered users ). If you find any errors ( broken links, can't load photos, etc.. ), Please let us know so we can fix it as soon as possible. And when you start a conversation or debate about a certain topic with other people, please do not offend them just because you don't like their opinions.
Concrete Construction Part 9 summary
You're reading Concrete Construction Part 9. This novel has been translated by Updating. Author: Halbert Powers Gillette and Charles Shattuck Hill already has 817 views.
It's great if you read and follow any novel on our website. We promise you that we'll bring you the latest, hottest novel everyday and FREE.
LightNovelFree.com is a most smartest website for reading novel online, it can automatic resize images to fit your pc screen, even on your mobile. Experience now by using your smartphone and access to LightNovelFree.com
- Related chapter:
- Concrete Construction Part 8
- Concrete Construction Part 10
RECENTLY UPDATED NOVEL
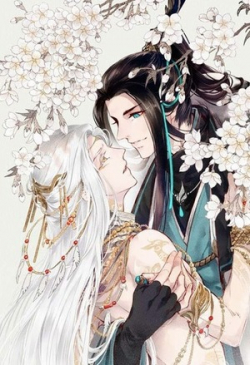
The Return Of The God Level Assassin
The Return Of The God Level Assassin Chapter 1176: YUNYUE VS FENGHUANG (XI) View : 394,544
I Love Destroying Worlds' Plot
I Love Destroying Worlds' Plot Chapter 1369: 19.28 Second Red Star - Never get involved when the Couple's Fight. View : 453,227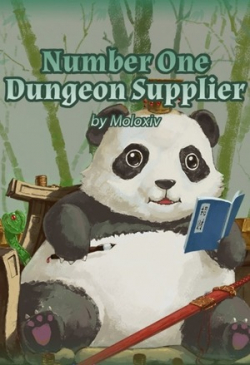
Number One Dungeon Supplier
Number One Dungeon Supplier Chapter 2108: Anti Celestial Compound View : 1,232,146