The Working of Steel Part 4
You’re reading novel The Working of Steel Part 4 online at LightNovelFree.com. Please use the follow button to get notification about the latest chapter next time when you visit LightNovelFree.com. Use F11 button to read novel in full-screen(PC only). Drop by anytime you want to read free – fast – latest novel. It’s great if you could leave a comment, share your opinion about the new chapters, new novel with others on the internet. We’ll do our best to bring you the finest, latest novel everyday. Enjoy!
For ball bearing steels, where intense hardness with great toughness and ready recovery from temporary deflection is required, chromium as an alloy offers the best solution.
Two per cent chromium steels; due to their very hard tough surface, are largely used for armor-piercing projectiles, cold rolls, crushers, drawing dies, etc.
The normal structure of chromium steels, with a very low carbon content is roughly pearlitic up to 7 per cent, and martensitic from 8 to 20 per cent; therefore, the greatest application is in the pearlitic zone or the lower percentages.
NICKEL-CHROMIUM
A combination of the characteristics of nickel and the characteristics of chromium, as described, should obviously give a very excellent steel as the nickel particularly affects the ferrite of the steel and the chromium the carbon. From this combination, we are able to get a very strong ferrite matrix and a very hard tough cement.i.te.
The strength of a strictly pearlitic steel over a pure iron is due to the pearlitic being a layer arrangement of cement.i.te running parallel to that of a pure iron layer in each individual grain. The ferrite _i.e._, the iron is increased in strength by the resistance offered by the cement.i.te which is the simple iron-carbon combination known to metallurgists as Fe3C. The cement.i.te, although adding to the tensile strength, is very brittle and the strength of the pearlite is the combination of the ferrite and cement.i.te. In the event of the cement.i.te being strengthened, as in the case of strictly chromium steels, an increased tensile strength is readily obtained without loss of ductility and if the ferrite is strengthened then the tensile strength and ductility of the metal is still further improved.
Nickel-chromium alloy represents one of the best combinations available at the present time. The nickel intensifies the physical characteristics of the chromium and the chromium has a similar effect on the nickel.
For case-hardening, nickel-chromium steels seem to give very excellent results. The carbon is very rapidly taken up in this combination, and for that reason is rather preferable to the straight nickel steel.
With the mutually intensifying action of chromium and nickel there is a most suitable ratio for these two alloys, and it has been found that roughly 2-1/2 parts of nickel to about 1 part of chromium gives the best results. Therefore, we have the standard types of 3.5 per cent nickel with 1.5 per cent chromium to 1.5 per cent nickel with 0.6 per cent chromium and the various intermediate types. This ratio, however, does not give the whole story of nickel-chromium combinations, and many surprising results have been obtained with these alloys when other percentage combinations have been employed.
VANADIUM
Vanadium has a very marked effect upon alloy steels rich in chromium, carbon, or manganese. Vanadium itself, when combined with steel very low in carbon, is not so noticeably beneficial as in the same carbon steel higher in manganese, but if a small quant.i.ty of chromium is added, then the vanadium has a very marked effect in increasing the impact strength of the alloy. It would seem that vanadium has the effect of intensifying the action of chromium and manganese, or that vanadium is intensified by the action of chromium or manganese.
Vanadium has the peculiar property of readily entering into solution with ferrite. If vanadium contained is considerable it also combines with the carbon, forming carbides. The ductility of carbon-vanadium steels is therefore increased, likewise the ductility of chrome-vanadium steels.
The full effect of vanadium is not felt unless the temperatures to which the steel is heated for hardening are raised considerably.
It is therefore necessary that a certain amount of "soaking" takes place, so as to get the necessary equalization. This is true of all alloys which contain complex carbides, i.e., compounds of carbon, iron and one or more elements.
Chrome-vanadium steels also are highly favored for case hardening.
When used under alternating stresses it appears to have superior endurance. It would appear that the intensification of the properties due to chromium and manganese in the alloy steel accounts for this peculiar phenomenon.
Vanadium is also a very excellent scavenger for either removing the harmful gases, or causing them to enter into solution with the metal in such a way as to largely obviate their harmful effects.
Chrome-vanadium steels have been claimed, by many steel manufacturers and users, to be preferable to nickel-chrome steels. While not wis.h.i.+ng to pa.s.s judgment on this, it should be borne in mind that the chrome-vanadium steel, which is tested, is generally compared with a very low nickel-chromium alloy steel (the price factor entering into the situation), but equally good results can be obtained by nickel-chromium steels of suitable a.n.a.lysis.
Where price is the leading factor, there are many cases where a stronger steel can be obtained from the chrome and vanadium than the nickel-chrome. It will be safe to say that each of these two systems of alloys have their own particular fields and chrome-vanadium steel should not be regarded as the sole solution for all problems, neither should nickel-chromium.
MANGANESE
Manganese adds considerably to the tensile strength of steel, but this is dependent on the carbon content. High carbon materially adds to the brittleness, whereas low-carbon, pearlitic-manganese steels are very tough and ductile and are not at all brittle, providing the heat-treating is correct. Manganese steel is very susceptible to high temperatures and prolonged heating.
In low-carbon pearlitic steels, manganese is more effective in increasing ultimate strength than is nickel; that is to say, a 0.45 carbon steel with 1.25 per cent manganese is as strong as a 0.45 carbon steel with 1.5 per cent nickel. The former steel is much used for rifle barrels, and in the heat-treated condition will give 80,000 to 90,000 lb. per square inch elastic limit, 115,000 to 125,000 lb. per square inch tensile strength, 23 per cent elongation, and 55 per cent reduction in area.
Manganese when added to steel has the effect of lowering the critical range; 1 per cent manganese will lower the upper critical point 60F. The action of manganese is very similar to that of nickel in this respect, only twice as powerful. As an instance, 1 per cent nickel would have the effect of lowering the upper critical range from 25 to 30F.
Low-carbon pearlitic-manganese steel, heat-treated, will give dynamic strength which cannot be equaled by low-priced and necessarily low-content nickel steels. In many instances, it is preferable to use high-grade manganese steel, rather than low-content nickel steel.
High-manganese steels or austenite manganese steels are used for a variety of purposes where great resistance to abrasion is required, the percentage of manganese being from 11 to 14 per cent, and carbon 1 to 1.5 per cent. This steel is practically valueless unless heat-treated; that is, heated to about yellow red and quenched in ice water. The structure is then austenite and the air-cooled structure of this steel is martensite. Therefore this steel has to be heated and very rapidly cooled to obtain the ductile austenite structure.
Manganese between 2 and 7 per cent is a very brittle material when the carbon is about 1 per cent or higher and is, therefore, quite valueless. Below 2 per cent manganese steel low in carbon is very ductile and tough steel.
The high-content manganese steels are known as the "Hadfield manganese steels," having been developed by Sir Robert Hadfield. Small additions of chrome up to 1 per cent increase the elastic limit of low-carbon pearlitic-manganese steels without affecting the steel in its resistance to shock, but materially decrease the percentage of elongation.
Vanadium added to low-carbon pearlitic manganese steel has a very marked effect, increasing greatly the dynamic strength and changing slightly the susceptibility of this steel to heat treatments, giving a greater margin for the hardening temperature. Manganese steel with added vanadium is most efficient when heat-treated.
TUNGSTEN
Tungsten, as an alloy in steel, has been known and used for a long time. The celebrated and ancient damascus steel being a form of tungsten-alloy steel. Tungsten and its effects, however, did not become generally realized until Robert Mushet experimented and developed his famous mushet steel and the many improvement made since that date go to prove how little Mushet himself understood the peculiar effects of tungsten as an alloy.
Tungsten acts on steel in a similar manner to carbon, that is, it increases its hardness, but is much less effective than carbon in this respect. If the percentage of tungsten and manganese is high, the steel will be hard after cooling in the air. This is impossible in a carbon steel. It was this combination that Mushet used in his well-known "air-hardening" steel.
The princ.i.p.al use of tungsten is in high-speed tool steel, but here a high percentage of manganese is distinctly detrimental, making the steel liable to fire crack, very brittle and weak in the body, less easily forged and annealed. Manganese should be kept low and a high percentage of chromium used instead.
Tools of tungsten-chromium steels, when hardened, retain their hardness, even when heated to a dark cherry red by the friction of the cutting or the heat arising from the chips. This characteristic led to the term "red-hardness," and it is this property that has made possible the use of very high cutting speeds in tools made of the tungsten-chromium alloy, that is, "high-speed" steel.
Tungsten steels containing up to 6 per cent do not have the property of red hardness any more than does carbon tool steel, providing the manganese or chromium is low.
When chromium is alloyed with tungsten, a very definite red-hardness is noticed with a great increase of cutting efficiency. The maximum red-hardness seems to be had with steels containing 18 per cent tungsten, 5.5 per cent chromium and 0.70 per cent carbon.
Very little is known of the actual function of tungsten, although a vast amount of experimental work has been done. It is possible that when the effect of tungsten with iron-carbon alloys is better known, a greater improvement can be expected from these steels.
Tungsten has been tried and is still used by some steel manufacturers for making punches, chisels, and other impact tools. It has also been used for springs, and has given very good results, although other less expensive alloys give equally good results, and are in some instances, better.
Tungsten is largely used in permanent magnets. In this, its action is not well understood. In fact, the reason why steel becomes a permanent magnet is not at all understood. Theories have been evolved, but all are open to serious questioning. The princ.i.p.al effect of tungsten, as conceded by leading authorities, is that it distinctly r.e.t.a.r.ds separation of the iron-carbon solution, removing the lowest recalescent point down to atmospheric temperature.
A peculiar property of tungsten steels is that if a heating temperature of 1,750F. is not exceeded, the cooling curves indicate but one critical point at about 1,350F. But when the heating temperature is raised above 1,850F., this critical point is nearly if not quite suppressed, while a lower critical point appears and grows enormously in intensity at a temperature between 660 and 750F.
The change in the critical ranges, which is produced by heating tungsten steels to over 1,850F., is the real cause of the red-hard properties of these alloys. Its real nature is not understood, and there is no direct evidence to show what actually happens at these high temperatures.
It may readily be understood that an alloy containing four essential elements, namely: iron, carbon, tungsten and chromium, is one whose study presents problems of extreme complexity. It is possible that complex carbides may be formed, as in chromium steels, and that compounds between iron and tungsten exist. Behavior of these combinations on heating and cooling must be better known before we are able to explain many peculiarities of tungsten steels.
MOLYBDENUM
Molybdenum steels have been made commercially for twenty-five years, but they have not been widely exploited until since the war. Very large resources of molybdenum have been developed in America, and the mining companies who are equipped to produce the metal are very active in advertising the advantages of molybdenum steels.
It was early found that 1 part molybdenum was the equivalent of from 2 to 2-1/2 parts of tungsten in tool steels, and magnet steels. It fell into disrepute as an alloy for high-speed tool steel, however, because it was found that the molybdenum was driven out of the surface of the tool during forging and heat treating.
Within the last few years it has been found that the presence of less than 1 per cent of molybdenum greatly enhances certain properties of heat-treated carbon and alloy steels used for automobiles and high-grade machinery.
In general, molybdenum when added to an alloy steel, increases the figure for reduction of area, which is considered a good measure of "toughness." Molybdenum steels are also relatively insensible to variations in heat treatment; that is to say, a chromium-nickel-molybdenum steel after quenching in oil from 1,450F.
may be drawn at any temperature between 900 and 1,100F. with substantially the same result (static tensile properties and hardness).
SILICON
Silicon prevents, to a large extent, defects such as gas bubbles or blow holes forming while steel is solidifying. In fact, steel after it has been melted and before it has been refined, is "wild"
and "ga.s.sy." That is to say, if it would be cast into molds it would froth up, and boil all over the floor. A judicious amount of silicon added to the metal just before pouring, prevents this action--in the words of the steel maker, silicon "kills" the steel.
If about 1.75 per cent metallic silicon remains in a 0.65 carbon steel, it makes excellent springs.
PHOSPHORUS
Phosphorus is one of the impurities in steel, and it has been the object of steel makers for years to eliminate it. On cheap grades of steel, not subject to any abnormal strain or stress, 0.1 per cent phosphorus is not objectionable. High phosphorus makes steel "cold short," i.e., brittle when cold or moderately warm.
SULPHUR
The Working of Steel Part 4
You're reading novel The Working of Steel Part 4 online at LightNovelFree.com. You can use the follow function to bookmark your favorite novel ( Only for registered users ). If you find any errors ( broken links, can't load photos, etc.. ), Please let us know so we can fix it as soon as possible. And when you start a conversation or debate about a certain topic with other people, please do not offend them just because you don't like their opinions.
The Working of Steel Part 4 summary
You're reading The Working of Steel Part 4. This novel has been translated by Updating. Author: Fred H. Colvin and K. A. Juthe already has 544 views.
It's great if you read and follow any novel on our website. We promise you that we'll bring you the latest, hottest novel everyday and FREE.
LightNovelFree.com is a most smartest website for reading novel online, it can automatic resize images to fit your pc screen, even on your mobile. Experience now by using your smartphone and access to LightNovelFree.com
- Related chapter:
- The Working of Steel Part 3
- The Working of Steel Part 5
RECENTLY UPDATED NOVEL

General, Your Wife Is Requesting Your Return Home For Farming
General, Your Wife Is Requesting Your Return Home For Farming Chapter 1458: Chapter 1206 (1): Untitled View : 694,514
My Girlfriend is a Zombie
My Girlfriend is a Zombie Chapter 829: Our Goal is to Tire Him Out! View : 2,285,346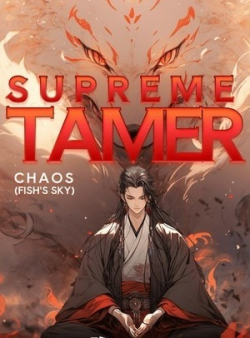
Supreme Tamer
Supreme Tamer Chapter 690: Chapter 559: Battle of the Three Great Main Pets View : 276,608
Trash of the Count's Family
Trash of the Count's Family Book 2: Chapter 249: Lunacy, Worship. And the Way (11) View : 813,061
Super Insane Doctor of the Goddess
Super Insane Doctor of the Goddess Chapter 1020: The Power of a True Dragon! View : 303,236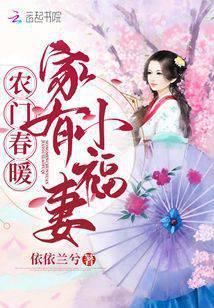