The Mechanism of Life Part 4
You’re reading novel The Mechanism of Life Part 4 online at LightNovelFree.com. Please use the follow button to get notification about the latest chapter next time when you visit LightNovelFree.com. Use F11 button to read novel in full-screen(PC only). Drop by anytime you want to read free – fast – latest novel. It’s great if you could leave a comment, share your opinion about the new chapters, new novel with others on the internet. We’ll do our best to bring you the finest, latest novel everyday. Enjoy!
The ions H^+ and OH^- are of special importance, since they are the ions of water, H_2O = H^+ + OH^-. The degree of {32} dissociation of pure water is but small. Water is, however, the most important of all the various agents in the chemical reactions of life, since a large number of organic substances are decomposed by water by a process of hydrolysis, and a vast number of organic substances are but combinations of carbon with the ions H^+ and OH^-, their diversity being due to variations in the relative proportions and grouping.
_The Chemical, Therapeutic, and Toxic Actions of Ions._--The chemical, therapeutic, antiseptic, and toxic actions of electrolytic solutions are almost exclusively due to ionization. Take, for instance, a solution of nitrate of silver in which the addition of chlorine produces a white precipitate of chloride of silver. This precipitate occurs only when the solution added is one such as NaCl, where the chlorine is present as the free ion Cl^-. No such precipitate is produced in a solution of chlorate of pota.s.sium or chloracetic acid, where the chlorine is entangled in the complex ion ClO_3 or C_2H_3ClO_2.
Since, then, the toxic and pharmacological properties of an electrolyte depend entirely on the ionic grouping, it behoves the physician and the biologist to study the structure and grouping of the ions in a molecule, rather than that of the atoms. Consider for a moment the totally different properties of the phosphides and the phosphates. The former are extremely toxic, while the latter are perfectly harmless. There is not the slightest a.n.a.logy between their actions on the living organism. On the other hand, all the phosphides produce the same toxic and therapeutic effects, whatever the cation with which they are united. Their toxic properties are derived from the presence of the free phosphorus ion P^{---}. The phosphates contain phosphorus in the same proportion as the phosphides, but this phosphorus is harmlessly entangled in the complex ion PO_4^{---}, whose properties are absolutely different from those of the ion P^{---}.
The above considerations apply equally to the chlorides and chlorates, the iodides and iodates, the sulphides and sulphates, and in general to all chemical salts. {33}
The question has an intimate bearing on practical pharmacology. When we prescribe a cacodylate or an amylarsinate, we are not prescribing an a.r.s.enical treatment whose effects can be compared with those of an a.r.s.enide, an a.r.s.enite, or an a.r.s.enate. This fact is sufficiently indicated by the difference in the toxic doses of the different salts. Each variety of a.r.s.enical ion has its own special physiological and therapeutic properties. We do not expect to obtain the results of a ferruginous treatment from the administration of a ferrocyanide or a ferricyanide. Both contain iron, it is true, but neither possess the properties of the cation Fe^{+++}, but rather those of the complex anion of which they form a part.
We have already said that most of the therapeutic, toxic, and caustic actions of an electrolyte are due to ionic action, and the substances can therefore have no toxic action unless they are dissociated. Many of the solvents employed in medicine, such as alcohol, glycerine, vaseline, and chloroform dissolve the electrolytes but do not dissociate them into ions, and these solutions therefore do not conduct electricity. Such solutions have no therapeutic action. With the absence of dissociation all the ionic toxic and caustic effects also disappear entirely, and only re-appear as the water of the tissue is able slowly to effect the necessary dissociation.
Carbolic acid dissolved in glycerine is hardly caustic and but very slightly toxic. We have met with several instances in which a tablespoonful of carbolized glycerine, in equal parts, has been swallowed without any ill effect, either caustic or toxic, whereas the same dose dissolved in water would have been fatal. This absence of dissociation has enabled the surgeon Menciere to inject carbolic and glycerine in equal proportions into the larger joints, the part being subsequently washed out with pure alcohol.
Thus by employing vaseline, oil, or glycerine as a solvent, and avoiding the access of water, we are able to use electrolytic antiseptics in very concentrated form. Their action is brought out very slowly, as the water of the organism effects the necessary dissociation of the electrolyte. {34}
Since all chemical, toxic, and therapeutic actions are ionic, they are proportional to the degree of ionic concentration, _i.e._ to the number of ions in a given volume. The only point of importance, that which determines their activity, whether chemical or therapeutic, is the degree of ionization or dissociation. For example, all acids have the same cation H^+. They have all identical properties, but they differ widely in the intensity of their action. There are weak acids such as acetic acid, and strong acids like sulphuric acid. The stronger acids are those which are more thoroughly dissociated, and in which the ion H^+ is very concentrated; whereas the feeble acids are but slightly dissociated, so that the ion H^+ is less concentrated.
Paul and Kronig have shown that the bactericidal action of different salts also varies with their degree of dissociation, _i.e._ with the concentration of the active ions. They made a series of observations on the bactericidal action of various salts of mercury, the b.i.+.c.hloride, the bibromide, and the bicyanide, on the spores of _Bacillus anthracis_. The following results were obtained from a comparison of solutions containing 1 gramme-molecule of the salt in 64 litres of water. With the b.i.+.c.hloride solution, after exposure to the solution for twenty minutes, only 7 colonies of the bacillus were developed. After exposure to a similar solution of the bibromide the number of colonies was 34. The antiseptic action of the b.i.+.c.hloride was therefore five times as great as that of the bibromide. The bicyanide of mercury, however, even when four times as concentrated, permitted the growth of an enormous number of colonies, showing that it had no appreciable antiseptic action whatever.
Nevertheless, the proportion of Hg is the same in all the solutions, and if there were any difference one would naturally expect that the ion Cy^- would be more toxic than Cl^- or Br^-. The real condition which varies in these solutions and determines their activity is the degree of dissociation. The whole of the antiseptic property resides in the ion Hg^{++}. This ion is very {35} concentrated in the highly dissociated solution HgCl_2, less concentrated in the less ionized solution HgBr_2, and exceedingly dilute in the HgCy_2, which is hardly ionized at all.
What is true of the bactericidal action of the salts of mercury is equally true of their therapeutic effect. It is a great mistake to estimate the medicinal activity of a solution of a salt of mercury, or indeed of any electrolytic solution, simply by its degree of molecular concentration. The important point is the degree of dissociation, which is the only true measure of its activity. In the intramuscular injection of mercury salts it is by no means a matter of indifference what salt we employ. A salt should be used such as the b.i.+.c.hloride or the biniodide, which is easily dissociated. Other salts are often employed because they occasion less pain at the site of injection; but the pain is a sign of the degree of activity of the preparation. The pain, it is true, may be avoided by using a salt which is less easily dissociated, or in which the mercury is bound up in a complex ion, but by so doing we diminish the efficacy of the remedy. It is moreover quite easy to diminish, or even entirely to suppress, the pain, by using a very dilute solution of an active ionized salt. A one-half per cent. or even one-quarter per cent. solution of the b.i.+.c.hloride or biniodide of mercury may be injected very slowly in sufficient quant.i.ty without producing the slightest discomfort. Local action depends entirely on ionic concentration. One drop of pure sulphuric acid will destroy the skin, whereas the same amount if diluted in a tumblerful of water will furnish a refres.h.i.+ng drink.
{36}
CHAPTER IV
COLLOIDS
As we have already seen, living organisms are formed essentially of liquids. These liquids are solutions of crystallizable substances or crystalloids, and non-crystallizable substances or colloids--a cla.s.sification which we owe to Graham.
The liquids are the most important const.i.tuents of a living organism, since they are the seat of all the chemical and physical phenomena of life. The junction of two liquids of different concentration is the arena in which takes place both the chemical transformation of matter and the correlative transformation of energy. In a former chapter we have pa.s.sed in review the cla.s.s of crystalloids, we will now turn our attention to the characteristic properties of colloids.
_Colloids._--Colloids differ from crystalloids in that they do not form crystals from solution, being completely amorphous when in the solid state.
The solution of a colloid solidifies in the same form which it possessed in the liquid state, the solvent being enclosed in the meshes of a sort of network formed by the solute. This form is approximately retained even after the water has evaporated by drying, the pa.s.sage from the liquid state of solution to the solid state being effected through a series of intermediary states, such as a clot, coagulum, or jelly. This pa.s.sage from the state of solution into a state of jelly is called coagulation. Some colloids, such as gelatine, coagulate with cold; while others, such as egg-alb.u.min, coagulate with heat. Some, like the caseine of milk, require the addition of certain chemical substances to set up coagulation; while still others, such as the fibrin of blood, appear to coagulate spontaneously. The physical phenomena of {37} coagulation are still but little understood. In some cases it is a reversible phenomenon, thus gelatine coagulated by cold is redissolved by heat; whereas with other colloids the process is irreversible, alb.u.min coagulated by heat is not redissolved on cooling.
Colloids in a state of coagulation have a vacuolar or sponge-like structure. The solvent is imprisoned in the vacuoles of the clot, and is expelled little by little by its retraction. Colloids diffused in water are usually called colloidal solutions, but they are not true solutions. Such a pseudo-solution of a colloid is called a "sol," while a colloid in a state of coagulation is called a "gel." Colloidal solutions spread but little, diffuse very slowly in the liquids of the body, and cannot penetrate organic membranes.
Colloidal solutions diffuse light, unlike crystalloid solutions, which are transparent. We all know how the trajectory of a beam of sunlight through a darkened room is rendered visible by the particles of dust. In the same way if a colloidal solution is illuminated by a transverse ray of light, the light is diffused by the molecules of the colloid in semi-solution, and the liquid appears faintly illuminated on a dark background. The light diffused by a colloidal solution is polarized, which shows that it is reflected light,
Siedentopf and Sigmondy have applied this principle of lateral illumination on a dark background to the construction of the ultra-microscope. With the aid of this instrument we may not only see, but count the particles in a colloidal solution, which is in reality merely a pseudo-solution or suspension, in contradistinction to the true solution of a crystalloid.
Colloidal solutions possess only a very feeble osmotic pressure. The lowering of the freezing point and the other corresponding constants are also quite insignificant. This arises from the fact that the molecules of a colloid are extremely large when compared with those of a crystalloid. For example let us take colloidal substance whose molecular weight is 2000. A solution containing 40 grammes per litre would have an osmotic pressure only one-fiftieth of that of a {38} solution of similar strength of a crystalloid whose molecular weight was 40.
Not only so, but on measuring the molecular concentration, the osmotic pressure, and the other constants of a colloidal solution, we find values even lower than those which we should expect from a consideration of its molecular weight. This is probably due to the tendency of a colloid to polymerization, i.e. to form groups or a.s.sociations of molecules. Suppose, for instance, that the molecules of a colloidal solution are aggregated into groups of ten. Since each group plays the part of a simple molecule, the osmotic pressure will be ten times less than that corresponding to the quant.i.ty of the solute present. Such a group of molecules is called by Naegeli a "micella."
Similar phenomena of aggregation may be observed in the molecules of many inorganic substances. The molecule of iodine, for example, is monatomic at 1200 C., but becomes diatomic at the ordinary temperature. Sulphur at 860 C. is a gas with a vapour density of 2.2, while at 500 C. its vapour density rises to 6.6. In both of these cases two or more molecules of the element have been condensed into one as a result of the fall of temperature.
We frequently find that two successive cryoscopic observations on the freezing point of the same colloidal solution will vary. This is due to the extreme sensitiveness of the micellae, which absorb or abandon their extra molecules under the slightest influence. This mobility in the const.i.tution of the micellae appears to be one of the princ.i.p.al causes of the peculiar properties of colloidal solutions.
The phenomenon of polymerization appears to be reversible. The micellae are formed under certain conditions, and are disintegrated when these conditions are removed. The osmotic pressure varies in the same manner, diminis.h.i.+ng with polymerization and augmenting with the disintegration of the micellae. One may easily understand what an important role is played by this alternate polymerization and disintegration in the phenomena of life.
Most colloidal substances are precipitated from their solutions by the addition of very small quant.i.ties of electrolytic {39} solutions.
Non-electrolytic solutions do not appear to provoke this precipitation.
This is not a chemical action, for an exceedingly small quant.i.ty of an electrolyte is able to precipitate an indefinite quant.i.ty of the colloid.
The precipitation is probably due to the electric charges carried by the dissociated ions of the electrolytes.
When an electric current is pa.s.sed through a colloid solution, the course of the molecules of the colloid is sometimes towards the cathode and sometimes towards the anode, according to the nature of the colloid and of the solvent. This displacement would appear to indicate a difference of electric potential between the molecules of the colloid and those of the solvent. Hardy has shown that in an alkaline solution the molecules of alb.u.min travel towards the anode, while in an acid solution they travel towards the cathode.
_Metallic Colloids._--Carey Lea and afterwards Crede succeeded in obtaining silver in colloidal solution by ordinary chemical means. Professor Bredig has introduced a more general method of obtaining a number of metals in colloidal solutions in a state of great purity. He causes an electric arc to pa.s.s between two rods of the metal immersed in distilled water. The cathode is thus pulverized into a very fine powder which rests in suspension in the liquid, const.i.tuting a colloidal solution. Bredig has in this way prepared sols of platinum, palladium, iridium, silver, and cadmium.
_Catalytic Properties of Colloids._--Catalysis is the property possessed by certain bodies of initiating chemical reaction. The ma.s.s of the catalyzing body has no definite proportion to that of the substances entering into the reaction, and the appearance of the catalyzer is in no way altered by the reaction.
Ostwald has shown that catalysis consists essentially in the acceleration or r.e.t.a.r.dation of chemical reactions which would take place without the action of the catalyzer, but more slowly.
Catalytic reactions are very numerous in chemistry. The inversion of sugar by acids, the etherization of alcohol by sulphuric acid, the decomposition of hydrogen peroxide by {40} platinum black are all instances of catalysis.
Fermentation by means of a soluble ferment or diastase, a phenomenon which may almost be called vital, is also a catalytic action. The action of pepsin, of the pancreatic ferment, of zymase, and of other similar ferments has a great a.n.a.logy with the purely physical phenomenon of catalysis. The diastases are all colloids, and so are many other catalyzers.
A catalyzer is a stimulus which excites a transformation of energy. The catalyzer plays the same role in a chemical transformation as does the minimal exciting force which sets free the acc.u.mulation of potential energy previous to its transformation into kinetic energy. A catalyzer is the friction of the match which sets free the chemical energy of the powder magazine.
Bredig has studied the catalytic decomposition of hydrogen peroxide by metallic colloids prepared by his electric method. He found that 1 atom-gramme of colloidal platinum gives a sensible catalytic effect when diluted with 70 million litres of water. Caustic soda and other chemical substances inhibit the catalytic action of colloidal platinum in the same way as they inhibit the fermenting action of diastase. The curve of decomposition of hydrogen peroxide by colloidal platinum may be compared with the curve of fermentation by emulsin. Both are equally affected by the addition of an alkali. Many other chemical and physical agents have a similar inhibitory action on the catalysis of colloidal metals and on diastasic fermentation. Thus a mere trace of sulphuretted hydrogen or hydrocyanic acid will paralyse the action of a colloidal metal, just as it does that of a ferment. This is what Bredig calls the poisoning of metallic ferments.
We may hope that the further study of catalysis, a purely physico-chemical phenomenon, may throw more light on the mechanism of diastasic fermentation, which is essentially a vital reaction.
It must not be forgotten that all cla.s.sification is artificial and arbitrary, and only to be used as long as it facilitates study. This observation is particularly applicable to the cla.s.sification of substances into crystalloids and colloids. {41} There is no sharp line between the two groups, the pa.s.sage is gradual, and it is impossible to say where one group ends and the other begins. Many colloids such as haemoglobin are crystallizable, and many crystallizable substances are coagulable. Many substances appear at one time in the crystalloid state and at another time in the colloidal state, so that instead of dividing substances into colloids and crystalloids, we should rather consider these expressions as denoting different phases a.s.sumed by the same substance.
In order to define clearly our various cla.s.ses and divisions, we are apt to exaggerate slight differences of properties or composition. We say that colloids have no osmotic pressure, whereas in fact the osmotic pressure of the colloids though feeble plays a very important part in the phenomena of life.
So in other departments of science--a factor which is almost infinitesimal may yet exercise a vast influence on the results. It is by infinitesimal variations of pressure, a thousandth of a millimetre or less, that we obtain the various degrees of penetration in the Rontgen rays.
The division into solutions and pseudo-solutions or suspensions is also an arbitrary one. A true solution is also a suspension of the molecules of the solute. There is no essential difference between a solution and a suspension, but only a difference in the size of the molecules, or agglomerations of molecules, in one case so small as to be transparent, and in the other case just big enough to diffuse light. There are moreover many properties common to colloidal solutions and suspensions of fine powders, such as kaolin, mastic, charcoal, or Indian ink. These particles in suspension are precipitated by solutions of electrolytes in a manner similar to the coagulation of colloids.
The surface of every liquid is covered by a very thin layer, a sort of membrane slightly differentiated from the rest of the liquid. This membrane may be a chemical one, a pellicular precipitate like that which is formed by the contact of two membranogenous liquids. On the other hand, the membrane may not differ from the subjacent liquid in chemical composition, but only in physical properties. If we {42} consider the molecules in the middle of a liquid, each molecule is subjected to the cohesive attraction of molecules on every side, attractions which neutralize one another. At the surface of the liquid, however, there are quite other conditions of equilibrium. There each molecule is drawn downwards towards the centre of the liquid, and there is no compensating attraction in an opposite direction. The resultant pressure is normal to the surface of the liquid, and is mechanically equivalent to an elastic membrane which tends to diminish the surface, and hence the volume of the liquid. We may therefore regard this surface tension as acting the part of a veritable physical membrane.
There is a still further differentiation of the surface of a liquid. When the liquid is not a simple one, but complex as in a solution, we find that the concentration of the solute is greater at the surface than in the interior. This is the so-called phenomenon of "adsorption," which is another cause for the production of a physical membrane covering the surface of a liquid.
Substances in a colloidal state have a great tendency to form these chemical or physical membranes at the point of contact between the colloidal solute and the solvent. This is probably the reason why the coagulum of a colloidal liquid usually presents a vacuolar or spongy structure.
{43}
CHAPTER V
DIFFUSION AND OSMOSIS
The Mechanism of Life Part 4
You're reading novel The Mechanism of Life Part 4 online at LightNovelFree.com. You can use the follow function to bookmark your favorite novel ( Only for registered users ). If you find any errors ( broken links, can't load photos, etc.. ), Please let us know so we can fix it as soon as possible. And when you start a conversation or debate about a certain topic with other people, please do not offend them just because you don't like their opinions.
The Mechanism of Life Part 4 summary
You're reading The Mechanism of Life Part 4. This novel has been translated by Updating. Author: Stephane Leduc already has 475 views.
It's great if you read and follow any novel on our website. We promise you that we'll bring you the latest, hottest novel everyday and FREE.
LightNovelFree.com is a most smartest website for reading novel online, it can automatic resize images to fit your pc screen, even on your mobile. Experience now by using your smartphone and access to LightNovelFree.com
- Related chapter:
- The Mechanism of Life Part 3
- The Mechanism of Life Part 5
RECENTLY UPDATED NOVEL
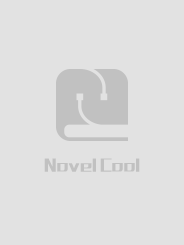
Reborn : Space Intelligent Woman
Reborn : Space Intelligent Woman Chapter 2098: Long Hui's Return View : 1,267,226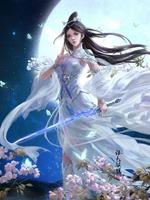
Journey To Become A True God
Journey To Become A True God Chapter 3591 Separating Ye Chen from Shen Xinyue and Lu Sansa View : 2,916,662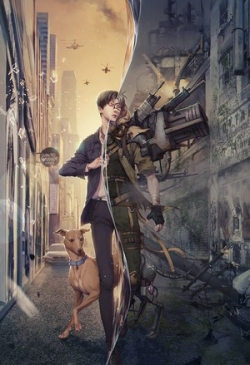
Global Game: AFK In The Zombie Apocalypse Game
Global Game: AFK In The Zombie Apocalypse Game Chapter 2537 The Third Item View : 2,865,900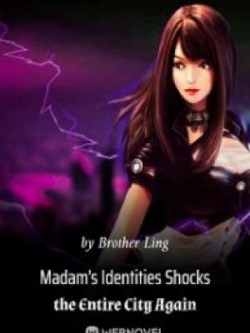
Madam's Identities Shocks The Entire City Again
Madam's Identities Shocks The Entire City Again Chapter 3343: The Ye Family Can Have No Grandchildren and Only Acknowledge This Granddaughter-in-law in the Future View : 2,561,496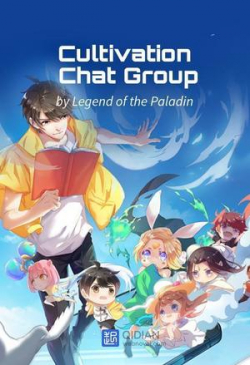