The Greatest Show On Earth, The Evidence For Evolution Part 3
You’re reading novel The Greatest Show On Earth, The Evidence For Evolution Part 3 online at LightNovelFree.com. Please use the follow button to get notification about the latest chapter next time when you visit LightNovelFree.com. Use F11 button to read novel in full-screen(PC only). Drop by anytime you want to read free – fast – latest novel. It’s great if you could leave a comment, share your opinion about the new chapters, new novel with others on the internet. We’ll do our best to bring you the finest, latest novel everyday. Enjoy!
Oster's model blastula gastrulating It gets even better. Oster and his colleagues tried the experiment, on their computer model, of lowering the 'firing threshold' of the contractile filaments. The result was a wave of inv.a.g.i.n.ation that went further, and actually pinched off a 'neural tube' (screenshots a to h, overleaf). It is important to understand what a model such as this really is. It is not an accurate representation of neurulation. Quite apart from the fact that it is two-dimensional and simplified in many other ways, the ball of cells that 'neurulated' (screenshot a a) was not a two-layered 'gastrula' as it should have been. It was the same blastula-like starting point as we had for the model of gastrulation above. It doesn't matter: models are not supposed to be totally accurate in every detail. The model still shows how easy it is to mimic various aspects of the behaviour of cells in an early embryo. The fact that the two-dimensional 'ball' of cells responded spontaneously to the stimulus even though the model is simpler than the real situation makes this a more powerful piece of evidence. It rea.s.sures us that the evolution of the various procedures of early embryonic development need not have been all that difficult. Note that it is the model that is simple, not the phenomenon that it demonstrates. That is the hallmark of a good scientific model.
Formation of 'neural tube' in Oster's model My purpose in expounding the Oster models has been to show the general kind kind of principle by which single cells can interact with each other to build a body, without any blueprint representing the whole body. Origami-like folding, Oster-style inv.a.g.i.n.ation and pinching off: these are just some of the simplest tricks for building embryos. Other more elaborate ones come into play later in embryonic development. For example, ingenious experiments have shown that nerve cells, when they grow out from the spinal cord, or from the brain, find their way to their end organ not by following any kind of overall plan but by chemical attraction, rather as a dog sniffs around to find a b.i.t.c.h in season. An early cla.s.sic experiment by the n.o.bel Prize-winning embryologist Roger Sperry ill.u.s.trates the principle perfectly. Sperry and a colleague took a tadpole and removed a tiny square of skin from the back. They removed another square, the same size, from the belly. They then regrafted the two squares, but each in the other's place: the belly skin was grafted on the back, and the back skin on the belly. When the tadpole grew up into a frog, the result was rather pretty, as experiments in embryology often are: there was a neat postage stamp of white belly skin in the middle of the dark, mottled back, and another neat postage stamp of dark mottled skin in the middle of the white belly. And now for the point of the story. Normally, if you tickle a frog on its back with a bristle, the frog will wipe the place with a foot, as if deterring an irritating fly. But when Sperry tickled his experimental frog on the white 'postage stamp' on its back, it wiped its belly! And when Sperry tickled it on the dark postage stamp on its belly, the frog wiped its back. of principle by which single cells can interact with each other to build a body, without any blueprint representing the whole body. Origami-like folding, Oster-style inv.a.g.i.n.ation and pinching off: these are just some of the simplest tricks for building embryos. Other more elaborate ones come into play later in embryonic development. For example, ingenious experiments have shown that nerve cells, when they grow out from the spinal cord, or from the brain, find their way to their end organ not by following any kind of overall plan but by chemical attraction, rather as a dog sniffs around to find a b.i.t.c.h in season. An early cla.s.sic experiment by the n.o.bel Prize-winning embryologist Roger Sperry ill.u.s.trates the principle perfectly. Sperry and a colleague took a tadpole and removed a tiny square of skin from the back. They removed another square, the same size, from the belly. They then regrafted the two squares, but each in the other's place: the belly skin was grafted on the back, and the back skin on the belly. When the tadpole grew up into a frog, the result was rather pretty, as experiments in embryology often are: there was a neat postage stamp of white belly skin in the middle of the dark, mottled back, and another neat postage stamp of dark mottled skin in the middle of the white belly. And now for the point of the story. Normally, if you tickle a frog on its back with a bristle, the frog will wipe the place with a foot, as if deterring an irritating fly. But when Sperry tickled his experimental frog on the white 'postage stamp' on its back, it wiped its belly! And when Sperry tickled it on the dark postage stamp on its belly, the frog wiped its back.What happens in normal embryonic development, according to Sperry's interpretation, is that axons (long 'wires', each one a narrow, tubular extension of a single nerve cell) grow questingly out from the spinal cord, sniffing like a dog for belly skin. Other axons grow out from the spinal cord, sniffing out back skin. And normally this gives the right result: tickles on the back feel as though they are on the back, while tickles on the belly feel as though they are on the belly. But in Sperry's experimental frog, some of the nerve cells sniffing out belly skin found the postage stamp of belly skin grafted on the back, presumably because it smelled right. And vice versa. People who believe in some sort of tabula rasa tabula rasa theory whereby we are all born with a blank sheet for a mind, and fill it in by experience must be surprised at Sperry's result. They would expect that frogs would learn from experience to feel their way around their own skin, a.s.sociating the right sensations with the right places on the skin. Instead, it seems that each nerve cell in the spinal cord is labelled, say, a belly nerve cell or a back nerve cell, even before it makes contact with the appropriate skin. It will later find its designated target pixel of skin, wherever it may be. If a fly were to crawl up the length of its back, Sperry's frog would presumably experience the illusion that the fly suddenly leaped from back to belly, crawled a little further, then instantaneously leaped to the back again. theory whereby we are all born with a blank sheet for a mind, and fill it in by experience must be surprised at Sperry's result. They would expect that frogs would learn from experience to feel their way around their own skin, a.s.sociating the right sensations with the right places on the skin. Instead, it seems that each nerve cell in the spinal cord is labelled, say, a belly nerve cell or a back nerve cell, even before it makes contact with the appropriate skin. It will later find its designated target pixel of skin, wherever it may be. If a fly were to crawl up the length of its back, Sperry's frog would presumably experience the illusion that the fly suddenly leaped from back to belly, crawled a little further, then instantaneously leaped to the back again.Experiments like this led Sperry to formulate his 'chemo-affinity' hypothesis, according to which the nervous system wires itself up not by following an overall blueprint but by each individual axon seeking out end organs with which it has a particular chemical affinity. Once again, we have small, local units following local rules. Cells in general bristle with 'labels', chemical badges that enable them to find their 'partners'. And we can go back to the origami a.n.a.logy to find another place where the labelling principle comes in useful. Human paper origami doesn't use glue, but it could. And the origami of the embryo, whereby animal bodies put themselves together, does indeed use something equivalent to glue. Glue s s, rather, because there are lots of them, and this is where labelling comes triumphantly into its own. Cells have a complicated repertoire of 'adhesion molecules' on their surfaces, whereby they stick to other cells. This cellular glueing plays an important role in embryonic development in all parts of the body. There is a significant difference from the glues that we are familiar with, however. For us, glue is glue is glue. Some glues are stronger than others, and some glues set faster than others, and some glues are more suitable for wood, say, while others work better for metals or plastics. But that's about it for variety among glues.Cell adhesion molecules are much more ingenious than that. More fussy, you could say. Unlike our artificial glues, which will stick to most surfaces, cell adhesion molecules bind only to particular other cell adhesion molecules of exactly the right kind. One cla.s.s of adhesion molecules in vertebrates, the cadherins, come in about eighty currently known flavours. With some exceptions, each of these eighty or so cadherins will bind only to its own kind. Forget glue for a minute: a better a.n.a.logy might be the children's party game where each child is a.s.signed an animal, and they all have to mill about the room making noises like their own allotted animals. Each child knows that only one other child has been a.s.signed the same animal as herself, and she has to find her partner by listening through the cacophony of farmyard imitations. Cadherins work like that. Perhaps, like me, you can dimly imagine how the judicious doping of cell surfaces with particular cadherins at strategic spots might refine and complicate the self-a.s.sembly principles of embryo origami. Note, once again, that this doesn't imply any kind of overall plan, but rather a piecemeal collection of local rules.
ENZYMES Having seen how whole sheets of cells play the origami game in shaping the embryo, let's now dive inside a single cell, where we'll find the same principle of self-folding and self-crumpling, but on a much smaller scale, the scale of the single protein molecule. Proteins are immensely important, for reasons that I must take time to explain, beginning with a teasing speculation to celebrate the unique importance of proteins. I love speculating on how weirdly different we should expect life to be elsewhere in the universe, but one or two things I suspect are universal, wherever life might be found. All life will turn out to have evolved by a process related to Darwinian natural selection of genes. And it will rely heavily on proteins or molecules which, like proteins, are capable of folding themselves up into a huge variety of shapes. Protein molecules are virtuosos of the auto-origamic arts, on a scale much smaller than that of the sheets of cells we have so far dealt with. Protein molecules are dazzling showcases of what can be achieved when local rules are obeyed on a local scale.Proteins are chains of smaller molecules called amino acids, and these chains, like the sheets of cells we have been considering, also fold themselves, in highly determined ways but on a much smaller scale. In naturally occurring proteins (this is one fact that will presumably be different on alien worlds) there are only twenty kinds of amino acid, and all proteins are chains strung together from just this repertoire of twenty, drawn from a much larger set of possible amino acids. Now for the auto-origami. Protein molecules, simply following the laws of chemistry and thermodynamics, spontaneously and automatically twist themselves into precisely shaped three-dimensional configurations I almost said 'knots' but, unlike hagfish (if I might impart a gratuitously inconsequential but engaging fact), proteins don't literally tie themselves in knots. The three-dimensional structure into which a protein chain folds and twists itself is the 'tertiary structure' that we briefly met when considering the self-a.s.sembly of viruses. Any given sequence of amino acids dictates a particular folding pattern. The amino-acid sequence, which itself is determined by the sequence of letters in the genetic code, determines the shape of the tertiary pattern.* The shape of the tertiary structure, in turn, has hugely important chemical consequences. The shape of the tertiary structure, in turn, has hugely important chemical consequences.The auto-origami by which protein chains fold and coil themselves is ruled by the laws of chemical attraction, and the laws determining the angles at which atoms bind to one another. Imagine a necklace of curiously shaped magnets. The necklace would not hang in a graceful catenary around a graceful neck. It would a.s.sume some other shape, becoming tangled up as the magnets latched on to each other and slotted into each other's nooks and crannies at various points along the length of the chain. Unlike the case of the protein chain, the exact shape of the tangle would not be predictable, because any magnet will attract any other. But it does suggest how chains of amino acids can spontaneously form a complicated knot-like structure, which may not look like a chain or a necklace.The details of how the laws of chemistry determine the tertiary structure of a protein are not yet fully understood: chemists can't yet deduce, in all cases, how a given sequence of amino acids will coil up. Nevertheless, there is good evidence that the tertiary structure is in principle in principle deducible from the sequence of amino acids. There's nothing mysterious about the phrase 'in principle'. n.o.body can predict how a die will fall, but we all believe it is wholly determined by precise details of how it is thrown, plus some additional facts about wind resistance and so on. It is a demonstrated fact that a particular sequence of amino acids always does coil up into a particular shape, or one of a discrete set of alternative shapes (see the long footnote opposite). And the important point for evolution the sequence of amino acids is itself fully determined, through implementation of the rules of the genetic code, by the sequence of (triplets of) 'letters' in a gene. Even though it is not easy for human chemists to predict what change in protein shape will result from a particular genetic mutation, it is still a fact that once a mutation has occurred, the resulting change of protein shape will be in principle predictable. The same mutant gene will reliably produce the same altered protein shape (or discrete menu of alternative shapes). And that is all that matters for natural selection. Natural selection doesn't need to understand why a genetic change has a certain consequence. It is sufficient that it does. If that consequence affects survival, the changed gene itself will stand or fall in the compet.i.tion to dominate the gene pool, whether or not we understand the exact route by which the gene affects the protein. deducible from the sequence of amino acids. There's nothing mysterious about the phrase 'in principle'. n.o.body can predict how a die will fall, but we all believe it is wholly determined by precise details of how it is thrown, plus some additional facts about wind resistance and so on. It is a demonstrated fact that a particular sequence of amino acids always does coil up into a particular shape, or one of a discrete set of alternative shapes (see the long footnote opposite). And the important point for evolution the sequence of amino acids is itself fully determined, through implementation of the rules of the genetic code, by the sequence of (triplets of) 'letters' in a gene. Even though it is not easy for human chemists to predict what change in protein shape will result from a particular genetic mutation, it is still a fact that once a mutation has occurred, the resulting change of protein shape will be in principle predictable. The same mutant gene will reliably produce the same altered protein shape (or discrete menu of alternative shapes). And that is all that matters for natural selection. Natural selection doesn't need to understand why a genetic change has a certain consequence. It is sufficient that it does. If that consequence affects survival, the changed gene itself will stand or fall in the compet.i.tion to dominate the gene pool, whether or not we understand the exact route by which the gene affects the protein.Given that protein shape is immensely versatile, and given that it is determined by genes, why is it so supremely important? Partly because some proteins have a direct structural role to play in the body. Fibrous proteins, such as collagen, join together in stout ropes, which we call ligaments and tendons. But most proteins are not fibrous. Instead, they fold themselves up into their own characteristic globular shape, complete with subtle dents, and this shape determines the protein's characteristic role as an enzyme enzyme, which is a catalyst catalyst.A catalyst is a chemical substance that speeds up, by as much as a billion or even a trillion times, a chemical reaction between other substances, while the catalyst itself emerges from the process unscathed and free to catalyse again. Enzymes, which are protein catalysts, are champions among catalysts because of their specificity specificity: they are very fussy about precisely which chemical reactions they speed up. Or perhaps we could say: chemical reactions in living cells are very fussy about which enzymes speed them up. Many reactions in cell chemistry are so slow that, without the right enzyme, for practical purposes they don't occur at all. But with the right enzyme they happen very fast, and can churn out products in bulk.Here's how I like to put it. A chemistry lab has hundreds of bottles and jars on its shelves, each containing a different pure substance: compounds and elements, solutions and powders. A chemist wis.h.i.+ng to perform a certain chemical reaction selects two or three bottles, takes a sample from each, mixes them in a test tube or a flask, perhaps applies heat, and the reaction takes place. Other chemical reactions that could take place in the lab don't, because the gla.s.s walls of the bottles and jars prevent the ingredients meeting. If you want a different chemical reaction, you mix different ingredients in a different flask. Everywhere there are gla.s.s barriers keeping the pure substances separate from one another in bottles or jars, and keeping the reacting combinations separate from one another in test tubes or flasks or beakers.The living cell, too, is a great chemistry lab, and it has a similarly large store of chemicals. But they aren't kept in separate bottles and jars on shelves. They are all mixed up together. It is as though a vandal, a chemical lord of misrule, entered the lab, seized all the bottles on all the shelves, and tipped them with anarchistic abandon into one great cauldron. Terrible thing to do? Well, it would be if they all reacted together, in all possible combinations. But they don't. Or if they do, the rate at which they react together is so slow that they might as well not be reacting at all. Except Except and this is the whole point if an enzyme is present. There is no need for gla.s.s bottles and jars to keep the substances apart because, to all intents and purposes, they are not going to react together anyway and this is the whole point if an enzyme is present. There is no need for gla.s.s bottles and jars to keep the substances apart because, to all intents and purposes, they are not going to react together anyway unless unless the right enzyme is present. The equivalent of keeping the chemicals in stoppered bottles until you want to mix a particular pair, say A and B, is to mix all the hundreds of substances up in a great witch's brew, but supply only the right enzyme to catalyse the reaction between A and B and no other combination. Actually, the metaphor of the anarchically inclined bottle-tipper goes too far. Cells do contain an infrastructure of membranes between which, and within which, chemical reactions go on. To some extent, these membranes play the role of gla.s.s part.i.tions between test tubes and flasks. the right enzyme is present. The equivalent of keeping the chemicals in stoppered bottles until you want to mix a particular pair, say A and B, is to mix all the hundreds of substances up in a great witch's brew, but supply only the right enzyme to catalyse the reaction between A and B and no other combination. Actually, the metaphor of the anarchically inclined bottle-tipper goes too far. Cells do contain an infrastructure of membranes between which, and within which, chemical reactions go on. To some extent, these membranes play the role of gla.s.s part.i.tions between test tubes and flasks.The point of this section of the chapter is that 'the right enzyme' achieves its 'rightness' largely through its physical shape (and that's important, because the physical shape is determined by genes, and it is genes whose variations are ultimately favoured or disfavoured by natural selection). Molecules aplenty are drifting and twisting and spinning through the soup that bathes the interior of a cell. A molecule of substance A might be happy to react with a molecule of substance B, but only if they happen to collide when facing in exactly the right direction, relative to each other. Crucially, that seldom happens unless unless the right enzyme intervenes. The enzyme's precise shape, the shape into which it folded itself like a magnetic necklace, leaves it pitted with cavities and dents, each one of which itself has a precise shape. Each enzyme has a so-called 'active site', which is usually a particular dent or pocket, whose shape and chemical properties confer upon the enzyme its specificity. The word 'dent' doesn't adequately convey the specificity, the precision, of this mechanism. Perhaps a better comparison is with an electric socket. In what my friend the zoologist John Krebs calls 'the great plug conspiracy', different countries around the world have irritatingly adopted different arbitrary conventions for plugs and sockets. British plugs won't fit American sockets, or French sockets, and so on. The active sites on the surface of protein molecules are sockets into which only certain molecules will fit. But whereas the great plug conspiracy runs to only half a dozen separate shapes around the world (quite enough to const.i.tute a continual annoyance to the traveller), the different kinds of sockets sported by enzymes are far more numerous. the right enzyme intervenes. The enzyme's precise shape, the shape into which it folded itself like a magnetic necklace, leaves it pitted with cavities and dents, each one of which itself has a precise shape. Each enzyme has a so-called 'active site', which is usually a particular dent or pocket, whose shape and chemical properties confer upon the enzyme its specificity. The word 'dent' doesn't adequately convey the specificity, the precision, of this mechanism. Perhaps a better comparison is with an electric socket. In what my friend the zoologist John Krebs calls 'the great plug conspiracy', different countries around the world have irritatingly adopted different arbitrary conventions for plugs and sockets. British plugs won't fit American sockets, or French sockets, and so on. The active sites on the surface of protein molecules are sockets into which only certain molecules will fit. But whereas the great plug conspiracy runs to only half a dozen separate shapes around the world (quite enough to const.i.tute a continual annoyance to the traveller), the different kinds of sockets sported by enzymes are far more numerous.Think of a particular enzyme, which catalyses the chemical combination of two molecules, P and Q, to make the compound PQ. One half of the active site 'socket' is just right for a molecule of type P to nestle into, like a jigsaw piece. The other half of the same socket is equally precisely shaped for a Q molecule to slot in facing exactly the right way to combine chemically with the P molecule that is already there. Sharing a dent, firmly held at just the right angle to each other by the matchmaking enzyme molecule, P and Q unite. The new compound, PQ, now breaks away into the soup, leaving the active dent in the enzyme molecule free to bring together another P and another Q. A cell may be filled with swarms of identical enzyme molecules, all working away like robots in a car factory, churning out PQ in the cellular equivalent of industrial quant.i.ties. Put a different enzyme into the same cell, and it will churn out a different product, perhaps PR, or QS or YZ. The end product is different, even though the available raw materials are the same. Other types of enzymes are concerned not with constructing new compounds, but with breaking down old ones. Some of these enzymes are involved in digesting food, and they are exploited, too, in 'biological' was.h.i.+ng powders. But, since this chapter is about the construction of embryos, we are here mostly concerned with constructive enzymes, which broker the synthesis of new chemical compounds. One such process is shown in action on colour page 12 page 12.A problem may have occurred to you. It's all very well to talk of jigsaw dents and sockets, highly specific active sites capable of speeding up a particular chemical reaction a trillionfold. But doesn't it sound too good to be true? How do enzyme molecules of exactly the right shape evolve from less perfect beginnings? What is the probability that a socket, shaped at random, will have just the right shape, and just the right chemical properties, to arrange a marriage between two molecules, P and Q, finessing their encounter at exactly the right angle? Not very great if you think 'finished jigsaw' or, indeed, if you think 'great plug conspiracy'. Instead, you have to think 'smooth gradient of improvement'. As so often when we are faced with the riddle of how complex and improbable things can arise in evolution, it is a fallacy to a.s.sume that the final perfection that we see today is the way it always was. Fully fas.h.i.+oned, highly evolved enzyme molecules achieve trillionfold speedups of the reactions they catalyse, and they do so by being beautifully crafted to exactly the right shape. But you don't need a trillionfold speedup in order to be favoured by natural selection. A millionfold will do nicely! So will a thousandfold. And even tenfold or twofold would be enough for natural selection to get an adequate grip. There is a smooth gradient of improvement in an enzyme's performance, all the way from almost no dent at all, through a crudely shaped dent, to a socket of exactly the right shape and chemical signature. 'Gradient' means that each step is a noticeable improvement, however slight, over the one before. And 'noticeable' for natural selection can mean an improvement smaller than the minimum that would be required for us to notice it.So, you see the way it works. Elegant! A cell is a versatile chemical factory, capable of spewing out ma.s.sive quant.i.ties of a wide variety of different substances, the choice being made by which enzyme is present. And how is that that choice made? By which gene is choice made? By which gene is turned on turned on. Just as the cell is a vat filled with lots of chemicals, only a minority of which react with each other, so every cell nucleus contains the entire genome, but with only a minority of genes turned on. When a gene is turned on in, say, a cell of the pancreas, its sequence of code letters directly determines the sequence of amino acids in a protein; and the sequence of amino acids determines (remember the image of the magnetic necklace?) the shape into which the protein folds itself; and the shape into which the protein folds itself determines the precisely shaped sockets that marry up substances drifting around in the cell. Every cell, with very few exceptions such as red blood corpuscles, which lack a nucleus, contains the genes for making all the enzymes. But in any one cell, only a few genes will be turned on at any one time. In, say, thyroid cells, the genes that make the right enzymes for catalysing the manufacture of thyroid hormone are turned on. And correspondingly for all the different kinds of cells. Finally, the chemical reactions that go on in a cell determine the way that cell is shaped and the way it behaves, and the way it partic.i.p.ates in origami-style interactions with other cells. So the whole course of embryonic development is controlled, via an intricate sequence of events, by genes. It is genes which determine sequences of amino acids, which determine tertiary structures of proteins, which determine the socket-like shapes of active sites, which determine cell chemistry, which determine 'starling-like' cell behaviour in embryonic development. So, differences in genes can, at the originating end of the complex chain of events, cause differences in the way embryos develop, and hence differences in the form and behaviour of adults. The survival and reproductive success of those adults then feeds back on the survival in the gene pool of the genes that made the difference between success and failure. And that is natural selection.
Cellular family tree of Caenorhabditis elegans Caenorhabditis elegans Embryology seems complicated is complicated but it is easy to grasp the important point, which is that we are dealing with local self-a.s.sembly processes all the way. It's a separate question, given that (almost) all the cells contain all the genes, how it is decided which genes are turned on in each different kind of cell. I must briefly deal with that now.
THEN WORMS SHALL TRY Whether or not a given gene is turned on in a given cell at a given time is determined, often via a cascade of other genes called switch genes or controller genes, by the chemical environment of the cell. Thyroid cells are quite different from muscle cells, and so on, even though their genes are the same. That's all very well, you may say, once the development of the embryo is under way, and the different kinds of tissues such as thyroid and muscle already exist. But every embryo starts out as a single cell. Thyroid cells and muscle cells, liver cells and bone cells, pancreas cells and skin cells, all are descended from a single fertilized egg cell, via a branching family tree. This is a cellular family tree going back no further than the moment of conception, nothing to do with the evolutionary tree going back millions of years, which keeps cropping up in other chapters. Let me show you, for example, the complete family tree of all 558 cells of a newly hatched larva of the nematode worm, Caenorhabditis elegans Caenorhabditis elegans (below: please pay close attention to every detail of this diagram). By the way, I don't know what this tiny worm did to earn its species name of (below: please pay close attention to every detail of this diagram). By the way, I don't know what this tiny worm did to earn its species name of elegans elegans, but I can think of a good reason why it might have deserved it retrospectively. I know that not all my readers like my digressions, but the research that has been done on Caenorhabditis elegans Caenorhabditis elegans is such a ringing triumph of science that you aren't going to stop me. is such a ringing triumph of science that you aren't going to stop me.Caenorhabditis elegans was chosen in the 1960s as an ideal experimental animal by the formidably brilliant South African biologist Sydney Brenner. He had recently completed his work, with Francis Crick and others at Cambridge, on cracking the genetic code, and was looking around for a new big problem to solve. His inspired choice, and his own pioneering research on its genetics and neuro-anatomy, has led to a worldwide community of was chosen in the 1960s as an ideal experimental animal by the formidably brilliant South African biologist Sydney Brenner. He had recently completed his work, with Francis Crick and others at Cambridge, on cracking the genetic code, and was looking around for a new big problem to solve. His inspired choice, and his own pioneering research on its genetics and neuro-anatomy, has led to a worldwide community of Caenorhabditis Caenorhabditis researchers that has grown into the thousands. It is only a bit of an exaggeration to say that we now know researchers that has grown into the thousands. It is only a bit of an exaggeration to say that we now know everything everything about about Caenorhabditis elegans Caenorhabditis elegans! We know its entire genome. We know exactly where every one of its 558 cells (in the larva; 959 in the adult hermaphroditic form, not counting reproductive cells) is in the body, and we know the exact 'family history' of every one of those cells, through embryonic development. We know of a large number of mutant genes, which produce abnormal worms, and we know exactly where the mutation acts in the body and the exact cellular history of how the abnormality develops. This little animal is known from start to finish, known inside out, known from head to tail and all stations in between, known through and through ('O frabjous day!'). Brenner was belatedly recognized with the n.o.bel Prize for Physiology in 2002, and a related species was named in his honour, Caenorhabditis brenneri Caenorhabditis brenneri. His regular column in the journal Current Biology Current Biology, under the byline 'Uncle Syd', is a model of intelligent and irreverent scientific wit as elegant as the worldwide research effort on C. elegans C. elegans that he inspired. But I do wish molecular biologists would talk to some zoologists (like Brenner himself) and learn not to refer to that he inspired. But I do wish molecular biologists would talk to some zoologists (like Brenner himself) and learn not to refer to Caenorhabditis Caenorhabditis as 'the' nematode, or even 'the' worm, as though there were no others. as 'the' nematode, or even 'the' worm, as though there were no others.Of course you can't read the names of the cell types at the bottom of the diagram (it would take seven pages to print the whole thing out legibly), but they say things like 'pharynx', 'intestinal muscle', 'body muscle', 'sphincter muscle', 'ring ganglion', 'lumbar ganglion'. The cells of all these types are literally cousins of one another: cousins by virtue of their ancestry within the lifetime of the individual worm. For example, I am looking at a particular body muscle cell called MSpappppa, which is a sibling of another body muscle cell, first cousin of two more body muscle cells, first cousin once removed of two more body muscle cells, second cousin of six pharynx cells, third cousin of seventeen pharynx cells . . . and so on. Isn't it amazing that we can actually use words like 'second cousin once removed', with the utmost precision and certainty, to refer to named and repeatably identifiable cells in an animal's body? The number of cell 'generations' that separates the tissues from the original egg is not that great. After all, there are only 558 cells in the body, and you can theoretically make 1,024 (2 to the power 10) in ten generations of cell splitting. The numbers of cell generations for human cells would be much larger. Nevertheless, you could in theory make a similar family tree for every one of your trillion-odd cells (as opposed to the 558 cells of a C. elegans C. elegans female larva), tracing each one's descent back to the one fertilized egg cell. In mammals, however, it is not possible to identify particular, repeatably named cells. In us, it is more a case of statistical populations of cells, whose details are different in different people. female larva), tracing each one's descent back to the one fertilized egg cell. In mammals, however, it is not possible to identify particular, repeatably named cells. In us, it is more a case of statistical populations of cells, whose details are different in different people.I hope my euphoric digression on the elegance of Caenorhabditis Caenorhabditis research has not distracted us too far from the point I was making about how cell types change in their shape and character as they branch away from one another in the embryonic family tree. At the branching point between a clone that is destined to become pharynx cells, and a 'cousin' clone that is destined to become ring ganglion cells, there has to be something to distinguish them, otherwise how would they know to turn on different genes? The answer is that, when the most recent common ancestor of the two clones divided, the two halves of the cell before division were different. So, when the cell divided, the two daughter cells, though identical in their genes (every daughter cell receives a full complement of genes), were not identical in the surrounding chemicals. And this meant that the same genes were not turned on which changed the fate of their descendants. The same principle applies right through embryology, including its very start. The key to differentiation, in all animals, is asymmetric cell division. research has not distracted us too far from the point I was making about how cell types change in their shape and character as they branch away from one another in the embryonic family tree. At the branching point between a clone that is destined to become pharynx cells, and a 'cousin' clone that is destined to become ring ganglion cells, there has to be something to distinguish them, otherwise how would they know to turn on different genes? The answer is that, when the most recent common ancestor of the two clones divided, the two halves of the cell before division were different. So, when the cell divided, the two daughter cells, though identical in their genes (every daughter cell receives a full complement of genes), were not identical in the surrounding chemicals. And this meant that the same genes were not turned on which changed the fate of their descendants. The same principle applies right through embryology, including its very start. The key to differentiation, in all animals, is asymmetric cell division.*Sir John Sulston and his colleagues traced each of the cells in the body of the worm back to one and only one of six founder cells we might even call them 'matriarch' cells called AB, MS, E, D, C and P4. In naming the cells, they used a neat notation that summarized the history of each one. Every cell's name begins with the name of one of those six founder cells, the one from which it is descended. Thereafter, its name is a string of letters, the initial letters of the direction of cell division that gave rise to it: anterior, posterior, dorsal, ventral, left, right. For example, Ca and Cp are the two daughters of matriarch C, the anterior and posterior daughter respectively. Notice that every cell has no more than two daughters (of which one may die). I am now looking at a particular body muscle cell, whose name, Cappppv, succinctly discloses its history: C had an anterior daughter, which had a posterior daughter, which had a posterior daughter, which had a posterior daughter, which had a posterior daughter, which had a ventral daughter, which is the body muscle cell in question. Every cell in the body is denoted by a comparable string of letters headed by one of the six founder cells. ABprpapppap, to take another example, is a nerve cell that sits in the ventral nerve cord running along the length of the worm. Needless to say, it is not necessary to take in the details. The beautiful point is that every single cell in the body has such a name, which totally describes its history during embryology. Every one of the ten cell divisions that gave rise to ABprpapppap, and every other cell, was an asymmetric division with the potential for different genes to be switched on in each of the two daughter cells. And in all animals that is the principle by which tissues differentiate, even though all their cells contain the same genes. Most animals, of course, have far more cells than Caenorhabditis' Caenorhabditis' 558, and their embryonic development is in most cases less rigidly determined. In particular, as Sir John Sulston kindly reminds me, and as I have already briefly mentioned, in a mammal the 'family trees' of our cells are different for every individual, whereas in 558, and their embryonic development is in most cases less rigidly determined. In particular, as Sir John Sulston kindly reminds me, and as I have already briefly mentioned, in a mammal the 'family trees' of our cells are different for every individual, whereas in Caenorhabditis Caenorhabditis they are almost identical (except in mutant individuals). Nevertheless, the principle remains the same. In any animal, cells differ from each other in different parts of the body, even though they are genetically identical, because of their history of asymmetric cell division during the short course of embryonic development. they are almost identical (except in mutant individuals). Nevertheless, the principle remains the same. In any animal, cells differ from each other in different parts of the body, even though they are genetically identical, because of their history of asymmetric cell division during the short course of embryonic development.Let us hear the conclusion of the whole matter. There is no overall plan of development, no blueprint, no architect's plan, no architect. The development of the embryo, and ultimately of the adult, is achieved by local rules implemented by cells, interacting with other cells on a local basis. What goes on inside cells, similarly, is governed by local rules that apply to molecules, especially protein molecules, within the cells and in the cell membranes, interacting with other such molecules. Again, the rules are all local, local, local. n.o.body, reading the sequence of letters in the DNA of a fertilized egg, could predict the shape of the animal it is going to grow into. The only way to discover that is to grow the egg, in the natural way, and see what it turns into. No electronic computer could work it out, unless it was programmed to simulate the natural biological process itself, in which case you might as well dispense with the electronic version and use the developing embryo as its own computer. This way of generating large and complex structures purely by the execution of local rules is deeply distinct from the blueprint way of doing things. If the DNA were some kind of linearized blueprint, it would be a relatively trivial exercise to program a computer to read the letters and draw the animal. But it would not be at all easy indeed, it might be impossible for the animal to have evolved in the first place.And now, so that this chapter on embryos should not end up as a mere digression in a book on evolution, I must return to the sincere dilemma of Haldane's questioner. Given that genes control processes of embryonic development rather than adult shape; given that natural selection like G.o.d doesn't build tiny wings, but embryology does; how does natural selection go to work on animals to shape their bodies and their behaviour? How does natural selection go to work on embryos, in other words, to rejig them so they become ever more proficient at building successful bodies, with wings, or fins, leaves or armour plating, stings or tentacles or whatever it takes to survive?Natural selection is the differential survival of successful genes rather than alternative, less successful genes in gene pools. Natural selection doesn't choose genes directly. Instead it chooses their proxies, individual bodies; and those individuals are chosen obviously and automatically and without deliberative intervention by whether they survive to reproduce copies of the very same genes. A gene's survival is intimately bound up with the survival of the bodies that it helps to build, because it rides inside those bodies, and dies with them. Any given gene can expect to find itself, in the form of copies of itself, riding inside a large number of bodies, both simultaneously in a population of contemporaries, and successively as generation gives way to generation. Statistically, therefore, a gene that tends, on average, to have a good effect on the survival prospects of the bodies in which it finds itself will tend to increase in frequency in the gene pool. So, on average, the genes that we encounter in a gene pool will tend to be those genes that are good at building bodies. This chapter has been about the procedures by which genes build bodies.Haldane's interlocutor found it implausible that natural selection could put together in, say, a billion years, a genetic recipe for building her. I find it plausible, although of course neither I nor anybody else can tell you the details of how it happened. The reason it is plausible is precisely that it is all done by local rules. In any one act of natural selection, the mutation that is selected has had in lots of cells and in lots of individuals in parallel a very simple simple effect on the shape into which a protein chain spontaneously coils up. This, in turn, through catalytic action, speeds up, say, a particular chemical reaction in all the cells in which the gene is turned on. This changes, perhaps, the rate of growth of the embryonic primordium of the jaw. And this has consequential effects on the shape of the whole face, perhaps shortening the muzzle and giving a more human and less 'ape-like' profile. Now, the natural selection pressures that favour or disfavour the gene can be as complicated as you like. They might involve s.e.xual selection, perhaps aesthetic choice of a high order by would-be s.e.xual partners. Or the change in jaw shape might have a subtle effect on the animal's ability to crack nuts, or its ability to fight rivals. Some hugely elaborate combination of selection pressures, conflicting and compromising with one another in bewildering complexity, can bear upon the statistical success of this particular gene, as it propagates itself through the gene pool. But the gene knows nothing of this. All it is doing, within different bodies and in successive generations, is rejigging a carefully sculpted dent in a protein molecule. The rest of the story follows automatically, in branching cascades of local consequences, from which, eventually, a whole body emerges. effect on the shape into which a protein chain spontaneously coils up. This, in turn, through catalytic action, speeds up, say, a particular chemical reaction in all the cells in which the gene is turned on. This changes, perhaps, the rate of growth of the embryonic primordium of the jaw. And this has consequential effects on the shape of the whole face, perhaps shortening the muzzle and giving a more human and less 'ape-like' profile. Now, the natural selection pressures that favour or disfavour the gene can be as complicated as you like. They might involve s.e.xual selection, perhaps aesthetic choice of a high order by would-be s.e.xual partners. Or the change in jaw shape might have a subtle effect on the animal's ability to crack nuts, or its ability to fight rivals. Some hugely elaborate combination of selection pressures, conflicting and compromising with one another in bewildering complexity, can bear upon the statistical success of this particular gene, as it propagates itself through the gene pool. But the gene knows nothing of this. All it is doing, within different bodies and in successive generations, is rejigging a carefully sculpted dent in a protein molecule. The rest of the story follows automatically, in branching cascades of local consequences, from which, eventually, a whole body emerges.Even more complicated than the selection pressures in the ecological, s.e.xual and social environments of the animals is the phantasmagoric network of influences that go on within and among the developing cells: influences of genes on proteins, genes on genes, proteins on the expression of genes, proteins on proteins; membranes, chemical gradients, physical and chemical guide rails in embryos, hormones and other mediators of action at a distance, labelled cells seeking others with identical or complementary labels. n.o.body understands the whole picture, and n.o.body needs to understand it in order to accept the exquisite plausibility of natural selection. Natural selection favours the survival in the gene pool of the genetic mutations responsible for making crucial changes in embryos. The whole picture emerges as a consequence of hundreds of thousands of small, local interactions, each one comprehensible in principle (although it may be too hard or too time-consuming to unravel in practice) to anyone with sufficient patience to examine it. The whole may be baffling and mysterious in practice, but there is no mystery in principle, either in embryology itself, or in the evolutionary history by which the controlling genes came to prominence in the gene pool. The complications acc.u.mulated gradually over evolutionary time: each step was only a tiny bit different from the one before, and each step was accomplished by a small, subtle change in an existing local rule. When you have a sufficient number of small ent.i.ties cells, protein molecules, membranes each at its own level obeying local rules and influencing others then the eventual consequence is dramatic. If genes survive or fail to survive as a consequence of their influence on such local ent.i.ties and their behaviour, natural selection of successful genes and the emergence of their successful products will inevitably follow. Haldane's questioner was wrong. It is not in principle difficult to make something like her.And, as Haldane said, it only takes nine months.
* I have been warned that 'All things bright and beautiful' will not necessarily strike my readers as nostalgically as it does me. It is an Anglican hymn for children written by Mrs C. F. Alexander in 1848, comfortably extolling the beauties of nature (and, in one verse, the political status quo) with the refrain, 'The Lord G.o.d made them all'. It is the subject of a splendid parody written by Eric Idle and sung by the Monty Python team: I have been warned that 'All things bright and beautiful' will not necessarily strike my readers as nostalgically as it does me. It is an Anglican hymn for children written by Mrs C. F. Alexander in 1848, comfortably extolling the beauties of nature (and, in one verse, the political status quo) with the refrain, 'The Lord G.o.d made them all'. It is the subject of a splendid parody written by Eric Idle and sung by the Monty Python team:All things dull and ugly All creatures short and squat All things rude and nasty The Lord G.o.d made the lot.Each little snake that poisons Each little wasp that stings He made their brutish venom He made their horrid wings.All things sick and cancerous All evil great and small, All things foul and dangerous The Lord G.o.d made them all.Each nasty little hornet Each beastly little squid Who made the spiky urchin?
Who made the sharks? He did!All things scabbed and ulcerous All pox both great and small Putrid, foul and gangrenous The Lord G.o.d made them all.
* Note for professionals at the interface between biologists and computer scientists: Charles Simonyi, who speaks with the authority of a distinguished software designer, put it as follows, after reading an early draft of this chapter: '. . . the recipe (of the eye, brain, blood, etc.) is much much simpler than the blueprint for the same (in terms of bits or base-pairs) so evolution would be literally impossible (in less than 10^100 years) especially because small variations in the blueprint would be unlikely to have any positive effect, whereas a variation in the recipe would.' Alluding to my own 'computer biomorphs' and 'arthromorphs' (see Chapter 2), Dr Simonyi goes on: 'The artificial creatures that you [programmed for Note for professionals at the interface between biologists and computer scientists: Charles Simonyi, who speaks with the authority of a distinguished software designer, put it as follows, after reading an early draft of this chapter: '. . . the recipe (of the eye, brain, blood, etc.) is much much simpler than the blueprint for the same (in terms of bits or base-pairs) so evolution would be literally impossible (in less than 10^100 years) especially because small variations in the blueprint would be unlikely to have any positive effect, whereas a variation in the recipe would.' Alluding to my own 'computer biomorphs' and 'arthromorphs' (see Chapter 2), Dr Simonyi goes on: 'The artificial creatures that you [programmed for The Blind Watchmaker The Blind Watchmaker and and Climbing Mount Improbable Climbing Mount Improbable] are all described by recipes, not by blueprint a blueprint would be just a jumble of vectors of black lines can you imagine trying to play evolution on them by varying the endpoints of the black lines one at a time or even two at a time?' As you'd expect from one described by Bill Gates, no less, as 'one of the great programmers of all time', this is exactly right for the computer biomorphs, and it is surely right for living things too.
* There is a risk that 'epigenesis' will be confused with 'epigenetics', a modish buzz-word now enjoying its fifteen minutes of fame in the biological community. Whatever 'epigenetics' might mean (and its enthusiasts cannot seem to agree even with themselves, let alone with each other), all I intend to say about it here is that it is not the same thing as epigenesis. There is a risk that 'epigenesis' will be confused with 'epigenetics', a modish buzz-word now enjoying its fifteen minutes of fame in the biological community. Whatever 'epigenetics' might mean (and its enthusiasts cannot seem to agree even with themselves, let alone with each other), all I intend to say about it here is that it is not the same thing as epigenesis.
* My medieval historian colleague Dr Christopher Tyerman confirms that this was indeed a myth that was invented in Victorian times for idealistic reasons, but that there was never a scintilla of truth in it. My medieval historian colleague Dr Christopher Tyerman confirms that this was indeed a myth that was invented in Victorian times for idealistic reasons, but that there was never a scintilla of truth in it.
* Inv.a.g.i.n.ate: 'fold inwards to form a hollow', 'turn or double back within itself' ( Inv.a.g.i.n.ate: 'fold inwards to form a hollow', 'turn or double back within itself' (Shorter Oxford English Dictionary).
* The craze died out, but I reintroduced it to the same school in the 1950s, whereupon it spread just like a second epidemic of the same disease. The craze died out, but I reintroduced it to the same school in the 1950s, whereupon it spread just like a second epidemic of the same disease.
* I am sorry I am at a loss to explain why the notochord gets an 'h', like a musical or mathematical chord, while the spinal cord doesn't, like a bit of string. I have always found it mysterious, and have even wondered whether it might represent some long-forgotten but fossilized mistake. Admittedly, the I am sorry I am at a loss to explain why the notochord gets an 'h', like a musical or mathematical chord, while the spinal cord doesn't, like a bit of string. I have always found it mysterious, and have even wondered whether it might represent some long-forgotten but fossilized mistake. Admittedly, the Oxford English Dictionary Oxford English Dictionary lists 'chord' as an alternative spelling for the string kind of cord, but the difference does seem queer given that the spinal cord and the notochord run the length of the embryonic body, one above the other. lists 'chord' as an alternative spelling for the string kind of cord, but the difference does seem queer given that the spinal cord and the notochord run the length of the embryonic body, one above the other.
* And that's a fascinating story in its own right, by the way. It has gripped my imagination ever since the great Cambridge physiologist Joseph Needham (a polymath who became even better known as the leading expert on the history of Chinese science) came to my school to demonstrate it, at the invitation of his nephew who happened to be our student teacher at the time: a boon of nepotism for which I remain grateful. Under Dr Needham's guidance, we peered at muscle fibres down our microscopes and watched them shorten, as if by magic, when we gave them a drop of ATP, adenosine triphosphate, the universal energy currency of the body. And that's a fascinating story in its own right, by the way. It has gripped my imagination ever since the great Cambridge physiologist Joseph Needham (a polymath who became even better known as the leading expert on the history of Chinese science) came to my school to demonstrate it, at the invitation of his nephew who happened to be our student teacher at the time: a boon of nepotism for which I remain grateful. Under Dr Needham's guidance, we peered at muscle fibres down our microscopes and watched them shorten, as if by magic, when we gave them a drop of ATP, adenosine triphosphate, the universal energy currency of the body.
* This statement needs an important reservation. The determination of the amino-acid sequence by genes is indeed absolute. But the determination of the three-dimensional shape by the one-dimensional amino-acid sequence is not absolute, and it really matters. There are some sequences of amino acids that are capable of coiling up into two alternative 3D shapes. The proteins called prions, for example, have two stable shapes. These are discrete alternatives without stable intermediates, in the same way as a light switch is stable in the up position and in the down position but nowhere in between. Such 'switch proteins' can be disastrous or they can be useful. In the case of prions they are disastrous. In 'mad cow disease', a useful protein in the brain (it's a normal const.i.tuent of cell membranes) happens to have an alternative form an alternative way to fold itself in auto-origami. The alternative form is normally never seen, but if it ever arises in one molecule it triggers neighbouring molecules to follow suit: they copy it and flip to the alternative form. Like a wave of falling dominoes, or like the irresponsible spreading of a rumour, the alternative prion form spreads through the brain, with disastrous results for the cow or the person in the case of CreutzfeldtJakob disease, or the sheep in the case of sc.r.a.pie. But sometimes molecules with the ability to auto-origami themselves into more than one alternative shape are useful. Without leaving the metaphor of the light switch we find a beautiful example. Rhodopsin, the protein in our eyes that is responsible for our sensitivity to light, has an embedded component called retinal (not itself a protein) which flips from its main stable configuration to its alternative configuration when hit by a photon of light. It then swiftly reverts, like a light switch on a cost-cutting timer, but meanwhile the flip has registered with the brain: 'Light detected at this pinpoint location here.' Jacques Monod's wonderful book, This statement needs an important reservation. The determination of the amino-acid sequence by genes is indeed absolute. But the determination of the three-dimensional shape by the one-dimensional amino-acid sequence is not absolute, and it really matters. There are some sequences of amino acids that are capable of coiling up into two alternative 3D shapes. The proteins called prions, for example, have two stable shapes. These are discrete alternatives without stable intermediates, in the same way as a light switch is stable in the up position and in the down position but nowhere in between. Such 'switch proteins' can be disastrous or they can be useful. In the case of prions they are disastrous. In 'mad cow disease', a useful protein in the brain (it's a normal const.i.tuent of cell membranes) happens to have an alternative form an alternative way to fold itself in auto-origami. The alternative form is normally never seen, but if it ever arises in one molecule it triggers neighbouring molecules to follow suit: they copy it and flip to the alternative form. Like a wave of falling dominoes, or like the irresponsible spreading of a rumour, the alternative prion form spreads through the brain, with disastrous results for the cow or the person in the case of CreutzfeldtJakob disease, or the sheep in the case of sc.r.a.pie. But sometimes molecules with the ability to auto-origami themselves into more than one alternative shape are useful. Without leaving the metaphor of the light switch we find a beautiful example. Rhodopsin, the protein in our eyes that is responsible for our sensitivity to light, has an embedded component called retinal (not itself a protein) which flips from its main stable configuration to its alternative configuration when hit by a photon of light. It then swiftly reverts, like a light switch on a cost-cutting timer, but meanwhile the flip has registered with the brain: 'Light detected at this pinpoint location here.' Jacques Monod's wonderful book, Chance and Necessity Chance and Necessity, is especially good on such bi-stable switch molecules.
* In In Caenorhabditis Caenorhabditis the original cell, called Z, has a front end which is different from its rear end, and this difference will come to represent the eventual fore-and-aft body axis anterior (front) and posterior (rear). When the cell divides, the anterior daughter cell, which is called AB, has more front-end substance than the posterior daughter cell, which is called P1, and this difference will be bootstrapped to make more differences down the line. AB is destined to give rise to well over half the cells of the body, including most of the nervous system, and I won't discuss it further. P1 has two children, again different from each other, called EMS (defining the ventral or belly side of the eventual worm) and P2 (defining the dorsal side). They are grandchildren of Z (remember, when I use words like 'children' and 'grandchildren', I am talking about cells within a developing embryo, not individual worms). EMS now has two children called E and MS, while P2 has two children called C and P3. E, MS, C and P3 are great-grandchildren of Z (the other great-grandchildren are descended from AB, and I am not writing them down, except to say that two of them, called ABal and ABpl, define the left side, and their cousins, ABar and Abpr, define the right side of the eventual worm). P3 has two children called D and P4, which are great-great-grandchildren of Z. MS and C also have children, but I shan't name them here. P4 is destined to give rise to the so-called germ line. The germ line consists of cells that are not involved in building the body, but instead are going to make the reproductive cells. Obviously there is no need to remember or take note of these cell names. The point is only that, although genetically identical to each other, they differ in their chemical nature, as a c.u.mulatively bootstrapped consequence of their history in the sequence of cell divisions within the embryo. the original cell, called Z, has a front end which is different from its rear end, and this difference will come to represent the eventual fore-and-aft body axis anterior (front) and posterior (rear). When the cell divides, the anterior daughter cell, which is called AB, has more front-end substance than the posterior daughter cell, which is called P1, and this difference will be bootstrapped to make more differences down the line. AB is destined to give rise to well over half the cells of the body, including most of the nervous system, and I won't discuss it further. P1 has two children, again different from each other, called EMS (defining the ventral or belly side of the eventual worm) and P2 (defining the dorsal side). They are grandchildren of Z (remember, when I use words like 'children' and 'grandchildren', I am talking about cells within a developing embryo, not individual worms). EMS now has two children called E and MS, while P2 has two children called C and P3. E, MS, C and P3 are great-grandchildren of Z (the other great-grandchildren are descended from AB, and I am not writing them down, except to say that two of them, called ABal and ABpl, define the left side, and their cousins, ABar and Abpr, define the right side of the eventual worm). P3 has two children called D and P4, which are great-great-grandchildren of Z. MS and C also have children, but I shan't name them here. P4 is destined to give rise to the so-called germ line. The germ line consists of cells that are not involved in building the body, but instead are going to make the reproductive cells. Obviously there is no need to remember or take note of these cell names. The point is only that, although genetically identical to each other, they differ in their chemical nature, as a c.u.mulatively bootstrapped consequence of their history in the sequence of cell divisions within the embryo. Sulston, who stayed at Cambridge after Brenner left for America, was another of the triumvirate who won the n.o.bel Prize for the Caenorhabditis Caenorhabditis work. Sulston went on to lead the British end of the official Human Genome Project, the American end of which was headed first by James Watson and later by Francis Collins. work. Sulston went on to lead the British end of the official Human Genome Project, the American end of which was headed
The Greatest Show On Earth, The Evidence For Evolution Part 3
You're reading novel The Greatest Show On Earth, The Evidence For Evolution Part 3 online at LightNovelFree.com. You can use the follow function to bookmark your favorite novel ( Only for registered users ). If you find any errors ( broken links, can't load photos, etc.. ), Please let us know so we can fix it as soon as possible. And when you start a conversation or debate about a certain topic with other people, please do not offend them just because you don't like their opinions.
The Greatest Show On Earth, The Evidence For Evolution Part 3 summary
You're reading The Greatest Show On Earth, The Evidence For Evolution Part 3. This novel has been translated by Updating. Author: Richard Dawkins already has 465 views.
It's great if you read and follow any novel on our website. We promise you that we'll bring you the latest, hottest novel everyday and FREE.
LightNovelFree.com is a most smartest website for reading novel online, it can automatic resize images to fit your pc screen, even on your mobile. Experience now by using your smartphone and access to LightNovelFree.com
- Related chapter:
- The Greatest Show On Earth, The Evidence For Evolution Part 2
- The Greatest Show On Earth, The Evidence For Evolution Part 4
RECENTLY UPDATED NOVEL
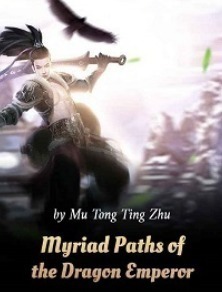
Myriad Paths of the Dragon Emperor
Myriad Paths of the Dragon Emperor Chapter 2849: 2848-Godking dies View : 1,360,585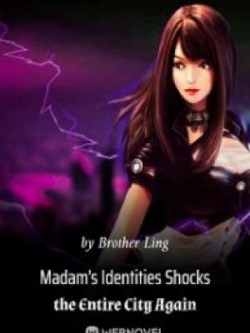
Madam's Identities Shocks The Entire City Again
Madam's Identities Shocks The Entire City Again Chapter 3342: Bullying Chen Chen Again View : 2,560,306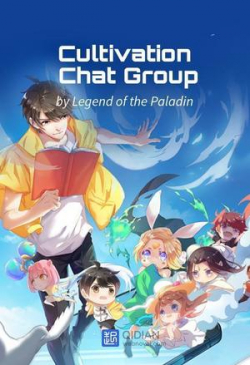
Cultivation Chat Group
Cultivation Chat Group Chapter 3056: Chapter 3054: Lady Kunna's Side Hustle View : 4,369,312
I Am Loaded With Passive Skills
I Am Loaded With Passive Skills Chapter 2698: The City Is Full of Snow (2) View : 4,177,361
Super Insane Doctor of the Goddess
Super Insane Doctor of the Goddess Chapter 932: End It! View : 257,682
Beauty and the Bodyguard
Beauty and the Bodyguard Chapter 11208: Chapter 11207: The Battle of the Three Kingdoms! View : 5,394,298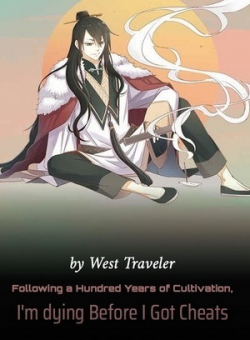
Following a Hundred Years of Cultivation, I'm dying Before I Got Cheats
Following a Hundred Years of Cultivation, I'm dying Before I Got Cheats Chapter 986: Fierce Battle, One Against Four View : 349,662
Warning : Providence the Beauty is Driven to Villainy
Warning : Providence the Beauty is Driven to Villainy Chapter 1193: After the Sea King overturned (7)_1 View : 255,061