Safe Food: Bacteria, Biotechnology, And Bioterrorism Part 3
You’re reading novel Safe Food: Bacteria, Biotechnology, And Bioterrorism Part 3 online at LightNovelFree.com. Please use the follow button to get notification about the latest chapter next time when you visit LightNovelFree.com. Use F11 button to read novel in full-screen(PC only). Drop by anytime you want to read free – fast – latest novel. It’s great if you could leave a comment, share your opinion about the new chapters, new novel with others on the internet. We’ll do our best to bring you the finest, latest novel everyday. Enjoy!
PART TWO
SAFETY AS A SURROGATETHE IRONIC POLITICS OF FOOD BIOTECHNOLOGYLATE IN THE FALL OF 2001, I ATTENDED A TUFTS UNIVERSITY conference on agricultural biotechnology sponsored by corporations such as Aventis (producer of StarLink corn) and Monsanto (producer of genetically modified cow growth hormone, corn, soybeans, and cotton). Speaker after speaker made the same three points: (1) the number of people in the world is increasing rapidly and food production must increase to keep them from starvation; (2) because the land available for growing food is limited, biotechnology-and only biotechnology-can increase food productivity; and (3) the main barrier to producing genetically modified foods is public doubt about their safety, particularly as expressed by unscientific activist groups such as Greenpeace. conference on agricultural biotechnology sponsored by corporations such as Aventis (producer of StarLink corn) and Monsanto (producer of genetically modified cow growth hormone, corn, soybeans, and cotton). Speaker after speaker made the same three points: (1) the number of people in the world is increasing rapidly and food production must increase to keep them from starvation; (2) because the land available for growing food is limited, biotechnology-and only biotechnology-can increase food productivity; and (3) the main barrier to producing genetically modified foods is public doubt about their safety, particularly as expressed by unscientific activist groups such as Greenpeace.1 Anyone not actively tracking the politics of food biotechnology might be surprised to learn that the chief impediment to eliminating world hunger is a consumer group best known for its opposition to nuclear weapons testing, but this topic is replete with such ironies. Anyone not actively tracking the politics of food biotechnology might be surprised to learn that the chief impediment to eliminating world hunger is a consumer group best known for its opposition to nuclear weapons testing, but this topic is replete with such ironies.To explain why the ironic politics of food biotechnology deserves attention in a book about food safety, we must begin with some definitions: biotechnology biotechnology and its synonym, and its synonym, genetic engineering genetic engineering, are processes by which scientists move genes (DNA) from one organism to another to transfer desired traits. Agricultural biotechnologists move genes from bacteria, viruses, or plants into food plants (the appendix explains how this is done). We call foods containing the new genes by a variety of equivalent terms: transgenic, bioengineered, genetically engineered (GE) transgenic, bioengineered, genetically engineered (GE), genetically modified (GM), genetically modified organisms (GMO) genetically modified (GM), genetically modified organisms (GMO), and, occasionally, the pejorative Franken-foods Franken-foods.2 These chapters refer to such foods interchangeably as These chapters refer to such foods interchangeably as genetically modified, genetically engineered genetically modified, genetically engineered, and transgenic transgenic.The speakers at the Tufts conference were intoning the mantra of the food biotechnology industry, the theoretical theoretical promise that its products will solve world food problems by creating a more abundant, more nutritious, and less expensive food supply. I emphasize theoretical because this promise is not yet realized; the industry is still in its infancy. The speakers were right to be concerned about public acceptance. The commercial products of food biotechnology have caused no end of controversy. In the United States, and particularly in Great Britain, people view the new foods with suspicion, often with dread and outrage. The results: boycotts, destruction of plantings ("ecoterrorism"), legal bans, and trade disputes. Such reactions reflect misgivings about the risks of technological manipulations of food, not only to human health, but also to the environment, to the world economy, and to society as a whole. They also reflect distrust of the motives of the food biotechnology industry and of the ability of government to regulate that industry. This sense of unease-specific for some, vague for others-translates most easily to a simple response: rejection. As people often tell me, "I don't want any GM in my food." promise that its products will solve world food problems by creating a more abundant, more nutritious, and less expensive food supply. I emphasize theoretical because this promise is not yet realized; the industry is still in its infancy. The speakers were right to be concerned about public acceptance. The commercial products of food biotechnology have caused no end of controversy. In the United States, and particularly in Great Britain, people view the new foods with suspicion, often with dread and outrage. The results: boycotts, destruction of plantings ("ecoterrorism"), legal bans, and trade disputes. Such reactions reflect misgivings about the risks of technological manipulations of food, not only to human health, but also to the environment, to the world economy, and to society as a whole. They also reflect distrust of the motives of the food biotechnology industry and of the ability of government to regulate that industry. This sense of unease-specific for some, vague for others-translates most easily to a simple response: rejection. As people often tell me, "I don't want any GM in my food."To industry officials and scientists who view risk through a science-based lens, statements like that are antiscientific and irrational. In the early 1990s, they characterized any any criticism of food biotechnology as ignorant, irresponsible, hysterical, or-my favorite-troglodyte, and as a prominent symptom of a new psychiatric disorder, biotechnophobia. criticism of food biotechnology as ignorant, irresponsible, hysterical, or-my favorite-troglodyte, and as a prominent symptom of a new psychiatric disorder, biotechnophobia.3 They lamented that well-funded activist groups were deliberately "interweaving political, societal and emotional issues . . . to delay commercialization and increase costs by supporting political, non-science-based regulation, unnecessary testing, and labeling of foods." They lamented that well-funded activist groups were deliberately "interweaving political, societal and emotional issues . . . to delay commercialization and increase costs by supporting political, non-science-based regulation, unnecessary testing, and labeling of foods."4 In that tradition, the Tufts conference speakers complained about the generous funding available to Greenpeace, another irony in light of the disparity between that group's resources and those of the agricultural biotechnology industry. In that tradition, the Tufts conference speakers complained about the generous funding available to Greenpeace, another irony in light of the disparity between that group's resources and those of the agricultural biotechnology industry.From its inception, food biotechnology has raised political, societal, and emotional issues: What are the risks of genetically modified foods? What are their benefits? How are risks and benefits distributed? Who makes decisions about them? How will genetically modified foods affect local, national, and international food systems and economies? How should the foods be regulated? Should they be labeled? And: Is it ethical to create such foods in the first place? The questions about risk can be answered scientifically, but the other questions are value-based and social social. Because questions about ethics and other social matters threaten the very foundation of food biotechnology, the industry and its supporters tend to restrict discussion to questions of safety. From a science-based perspective, if genetically modified foods are safe, there is no sensible reason for regulating, labeling, or opposing them.The focus on science, safety, and risk obscures the social issues, particularly those having to do with the distribution of economic benefits. Food biotechnology is a huge business, and huge profits are at stake. To survive, the industry must make products that farmers or the public will buy. Politics enters the picture because other stakeholders in the food system have different agendas and hold different values. Scientists want to work on challenging problems that might produce health or economic gains, and, as a necessary benefit, research funding. Government regulators want to ensure that foods are safe, but they also want to avoid congressional intervention and industry lawsuits. As consumers, we all want food that is safe (or safe enough), but many of us also are concerned about social issues. Food biotechnology is political because basic questions-Who benefits? Who decides? Who controls?-require societal resolution and cannot be decided solely by the methods of science.The debates about food biotechnology are especially complicated because the science itself is so complicated. That most people cannot understand the science behind genetically modified foods is a given. But anyone, trained in science or not, can grasp whether democratic political processes are at work in making decisions about these foods. We will see how questions of democracy-and the lack of an inst.i.tutional venue for debating the social implications of food biotechnology-underlie much of the distrust of the industry and its government regulators. The desire for democratic processes and the trust they inspire explain why the lack of labeling of genetically modified foods is such a critical point of debate. Labeling places the power to make decisions in the hands of consumers, not the industry.Although the safety of genetically modified foods is an important issue, it is not the only one of interest. But because safety appears to be the only legitimate legitimate ground for criticism, it acts as a surrogate for concerns about democratic processes and social implications. The StarLink corn affair is an example of the use of safety as a surrogate; the arguments focused on allergenicity (science), but the real issues had to do with the company's control over the food supply and evasion of democratic processes of government oversight (social values). The politics of food biotechnology matter because the disputes s.h.i.+ft attention away from the underlying issues. If, for example, the roots of world hunger lie in poverty, we should be debating options for redressing economic imbalances. If we want to meet the food needs of the twenty-first century, we ought to be considering a broad range of alternatives, among which biotechnology may or may not be the best. Social problems are manifestly difficult to address, as their causes are multiple and complex. It is understandable that we might find simple, "reductionist" approaches to such problems-like genetically engineering vitamins into rice-preferable to the messy business of political action to address world poverty. ground for criticism, it acts as a surrogate for concerns about democratic processes and social implications. The StarLink corn affair is an example of the use of safety as a surrogate; the arguments focused on allergenicity (science), but the real issues had to do with the company's control over the food supply and evasion of democratic processes of government oversight (social values). The politics of food biotechnology matter because the disputes s.h.i.+ft attention away from the underlying issues. If, for example, the roots of world hunger lie in poverty, we should be debating options for redressing economic imbalances. If we want to meet the food needs of the twenty-first century, we ought to be considering a broad range of alternatives, among which biotechnology may or may not be the best. Social problems are manifestly difficult to address, as their causes are multiple and complex. It is understandable that we might find simple, "reductionist" approaches to such problems-like genetically engineering vitamins into rice-preferable to the messy business of political action to address world poverty.This part of the book deals with how and why the safety of genetically modified foods became a surrogate for concerns about larger social issues.5 In telling this story, these chapters continue many of the themes noted earlier: industry promotion of economic self-interest at the expense of health and safety, the industry's political efforts to prevent imposition of regulatory controls and labeling requirements, the fragmentation and consequent weakness of government oversight, the imbalance in power between corporate and public interests, and the use of science as a rationale for self-interested actions. In telling this story, these chapters continue many of the themes noted earlier: industry promotion of economic self-interest at the expense of health and safety, the industry's political efforts to prevent imposition of regulatory controls and labeling requirements, the fragmentation and consequent weakness of government oversight, the imbalance in power between corporate and public interests, and the use of science as a rationale for self-interested actions.The discussion of these themes begins in chapter 5 chapter 5 with an introduction to the food biotechnology industry-its methods, promises, and realities. Much of the chapter is devoted to a discussion of the "poster child" for the benefits of genetically modified foods, Golden Rice, a rice bioengineered to contain beta-carotene, a precursor of vitamin A. with an introduction to the food biotechnology industry-its methods, promises, and realities. Much of the chapter is devoted to a discussion of the "poster child" for the benefits of genetically modified foods, Golden Rice, a rice bioengineered to contain beta-carotene, a precursor of vitamin A. Chapter 6 Chapter 6 evaluates the benefits claimed for genetically modified foods, as well as their safety risks: allergenicity, antibiotic resistance, and environmental impact. In evaluates the benefits claimed for genetically modified foods, as well as their safety risks: allergenicity, antibiotic resistance, and environmental impact. In chapter 7 chapter 7, I discuss the politics of government oversight of genetically modified foods and describe how the industry convinced federal regulatory agencies to use a strictly science-based approach to risk evaluation, thereby allowing companies to plant first, then then deal with problems (rather than requiring premarket testing). deal with problems (rather than requiring premarket testing). Chapter 8 Chapter 8 focuses on the important societal issues that spark protests against genetically modified foods: consumer choice at the marketplace (labeling), inequities in owners.h.i.+p of plant resources (intellectual property rights or "biopiracy"), the accidental movement of transgenes into conventional crops ("genetic pollution"), and corporate control of the food supply (globalization). Overall, these chapters provide an a.n.a.lysis of where the issues raised by food biotechnology stand today, and how industry, scientists, government, and the public might deal with the ongoing disputes about genetically modified foods. focuses on the important societal issues that spark protests against genetically modified foods: consumer choice at the marketplace (labeling), inequities in owners.h.i.+p of plant resources (intellectual property rights or "biopiracy"), the accidental movement of transgenes into conventional crops ("genetic pollution"), and corporate control of the food supply (globalization). Overall, these chapters provide an a.n.a.lysis of where the issues raised by food biotechnology stand today, and how industry, scientists, government, and the public might deal with the ongoing disputes about genetically modified foods.
CHAPTER 5.
PEDDLING DREAMS.
PROMISES VERSUS REALITY.
BIOTECHNOLOGY COMPANIES HAD BEEN WORKING ON AGRICULtural projects for 10 years or more when, in 1992, I received a last-minute invitation to talk about the labeling of genetically modified foods at a conference organized by Public Voice, a consumer advocacy group for food and health policy in Was.h.i.+ngton, DC. As a trained molecular biologist-though a long lapsed one-I was intrigued by the possibilities of the technology. I had not been following the field very closely and was puzzled about why an advocacy group might be concerned about labeling products that were still hypothetical. As it happened, I was not unprepared to address the question. For teaching purposes, I routinely collect scientific articles and newspaper clippings on nutrition topics, and I had acc.u.mulated a thick file on food biotechnology. The invitation provided an excuse to see what was in it.
The file surprised me. It immediately revealed that the industry's exciting promise to solve world food problems had little to do with the reality of its research and development efforts. Instead, companies were working on crop products most likely to generate returns on investment. Furthermore, industry leaders seemed to view the public not as an enthusiastic partner in enhancing the food supply but rather as a hostile force threatening their economic viability. The industry and its supporters in science, government, and business framed public questions about the safety or other consequences of food biotechnology as irrational challenges by scientifically illiterate consumers. I could not evaluate their science-based contentions that the techniques were inherently safe and the foods no different from those produced by conventional genetic crosses, however, as none had yet come to market.
Since then, the situation has changed in some ways but not in others. Once the Food and Drug Administration (FDA) approved the marketing of genetically modified foods in 1994, the production of these foods grew rapidly. By 2001, genetically modified varieties accounted for 26% of the corn and 68% of the soybeans planted in the United States as well as 69% of the cotton (the source of cottonseed oil for animal feed). Manufacturers were using ingredients made from transgenic corn and soybeans in 60% or more of processed foods on supermarket shelves-baby formulas, drink mixes, m.u.f.fin mixes, fast foods, and, as we have seen, taco sh.e.l.ls. Early in the twenty-first century, it is not possible to keep genetically modified foods out out of the food supply. of the food supply.1 What should we, as citizens and consumers, make of this situation? This chapter establishes a basis for answering that question by examining the promises of the food biotechnology industry-what it could could do-in comparison to the reality of its products and actions. do-in comparison to the reality of its products and actions.
THE THEORETICAL PROMISES.
In theory, if not yet in practice, food biotechnology holds much promise for addressing world food problems, most notably the overall shortfall in food production expected early in the twenty-first century. By some estimates, the global demand for rice, wheat, and maize will increase by 40% above current levels as early as 2020.2 To feed an increasing population on a constant area of arable land, the land must produce much more food-and do so without irreversibly damaging the environment. No technical barriers-again, in theory-prevent the use of genetic manipulations to improve the quant.i.ty and quality of the food supply, increase its safety, reduce the use of harmful pesticides and agricultural chemicals, and reduce food costs. To feed an increasing population on a constant area of arable land, the land must produce much more food-and do so without irreversibly damaging the environment. No technical barriers-again, in theory-prevent the use of genetic manipulations to improve the quant.i.ty and quality of the food supply, increase its safety, reduce the use of harmful pesticides and agricultural chemicals, and reduce food costs. Table 11 Table 11 lists examples of the stunning range of potentially beneficial applications of food biotechnology that are now available or under investigation. lists examples of the stunning range of potentially beneficial applications of food biotechnology that are now available or under investigation. Figure 11 Figure 11 ill.u.s.trates a cartoonist's somewhat ironic view of such possibilities. ill.u.s.trates a cartoonist's somewhat ironic view of such possibilities.
These applications could increase world food production, especially given the conditions of poor climate and environmental degradation characteristic of many developing countries, and they also could improve the nutritional quality of indigenous food plants on which so many populations depend. The potential for such improvements explains why industry leaders refer to food biotechnology as "the most important scientific tool to affect the food economy in the history of mankind," "the single most promising approach to feeding a growing world population while reducing damage to the environment," and an innovation that will "create miracles to help us feed a hungry world efficiently and economically."3 Such statements promise that food biotechnology will improve the food supply more effectively than conventional genetic techniques-those that involve selecting plants with desired traits, cross-pollinating them with related stock, and selecting and growing the progeny for many generations under field conditions. As this chapter explains, food biotechnologists consider such methods to be slow and imprecise and far inferior to their own. Such statements promise that food biotechnology will improve the food supply more effectively than conventional genetic techniques-those that involve selecting plants with desired traits, cross-pollinating them with related stock, and selecting and growing the progeny for many generations under field conditions. As this chapter explains, food biotechnologists consider such methods to be slow and imprecise and far inferior to their own.
TABLE 11. Theoretical and current applications of food biotechnology Food Plants (for human use) Improve flavor, texture, or freshness.
Increase levels of vitamins, protein, and other nutrients.
Increase production of chemicals such as sugars, waxes, or nutritionally important components.
Decrease levels of caffeine or other undesirable chemical substances.
Reduce saturated fatty acids in plant seed oils.
Produce drugs such as antibiotics, vaccines, or contraceptives.
Crop Plants (mainly for animal feed) Introduce herbicide resistance to improve weed control.
Permit growth with minimal use of fertilizers, pesticides, or water.
Increase resistance to damage by insect, fungal, viral, or other microbial pests.
Increase resistance to "stress" by frost, heat, salt, or heavy metals.
Permit fixation of atmospheric nitrogen.
Increase grain content of scarce amino acids.
Food Animals (for human use) Increase the efficiency of growth and reproduction.
Strengthen disease resistance.
Develop veterinary vaccines and diagnostic tests.
Increase milk production.
Produce milk containing pharmaceuticals.
The promise that food biotechnology will provide food for a hungry world, however, has yet to be fulfilled and is unlikely to be realized in the immediate future. Many of the applications listed in table 11 table 11 pose technical problems of formidable complexity. It is not easy to identify genes for desired traits, isolate them, insert them into plants, and provide the additional molecular components needed to make them function properly. The slow progress of biotechnology in addressing world hunger does not imply that this problem cannot be solved; given sufficient time, commitment, and funding support, the technical barriers could well be overcome. pose technical problems of formidable complexity. It is not easy to identify genes for desired traits, isolate them, insert them into plants, and provide the additional molecular components needed to make them function properly. The slow progress of biotechnology in addressing world hunger does not imply that this problem cannot be solved; given sufficient time, commitment, and funding support, the technical barriers could well be overcome.4 [image]
FIGURE 11. This political commentary, "Genetically Modified Specials," appeared as an "op-art" opposite the editorial page of the New York Times New York Times, July 15, 2000. ( 2000 Jesse Gordon and Knickerbocker Design. Reprinted with permission.) Technical problems, therefore, are a temporary barrier and are not the most important one. Instead, the main barrier to producing more food for the developing world is economic. Food biotechnology is a business, and businesses must generate returns on investment. In the food biotechnology business, economic aims (the reality) compete with humanitarian aims (the promises). These purposes conflict: one goal is to produce more and better food for an increasing population, but another is to produce foods with a compet.i.tive advantage in today's global marketplace-particularly "value-added" foods processed in ways that generate benefits for consumers and higher profits for manufacturers.5 Although genetically modified foods might well be expected to meet both goals, they often do not. Like all industries, this one serves investors who demand rapid returns, and financial considerations inevitably influence decisions related to product development. The business imperatives explain why the industry continues to view legitimate public questions about the use, safety, or social consequences of particular products as threats to the entire biotechnology enterprise. Without substantial changes to the economic realities of food biotechnology, its feed-the-world potential remains an unfulfilled promise. Although genetically modified foods might well be expected to meet both goals, they often do not. Like all industries, this one serves investors who demand rapid returns, and financial considerations inevitably influence decisions related to product development. The business imperatives explain why the industry continues to view legitimate public questions about the use, safety, or social consequences of particular products as threats to the entire biotechnology enterprise. Without substantial changes to the economic realities of food biotechnology, its feed-the-world potential remains an unfulfilled promise.
THE ECONOMIC REALITIES.
If food biotechnology companies are primarily businesses, then their primary concern is to recover the costs of research and development and to maximize returns on investment. Research costs can be high; it takes years and hundreds of millions of dollars to bring a genetically engineered food to market. Nevertheless, even before the FDA approved the first such food for production, business a.n.a.lysts viewed the industry as one with a huge market potential. In 1992, they predicted that the value of the industry would increase to at least $50 billion by the year 2000. As late as 1998, some were predicting that worldwide sales could exceed $300 billion by 2010. These predictions were overly optimistic, but food biotechnology is still big business. Worldwide sales of genetically modified crops rose from $1.6 billion in 1998 to about $2.2 billion in 1999, and are now expected to rise to $25 billion by 2010.6 Regardless of the accuracy of such estimates, the rapid expansion of the food biotechnology industry is impressive. By 1998, about 1,400 companies had invested more than $110 billion in agricultural biotechnology, and the FDA had approved about 50 food products for marketing. By 2001, genetically engineered crops were growing on at least 109 million acres throughout the world, a 25-fold expansion just since 1996. Although 80% of the acres were in North America, Argentina, and China, 10 other countries also had substantial plantings and more than 40 countries permitted field trials of one crop or another, most intended for animal feed.7 Despite the recent decline in planting of genetically engineered corn that occurred as a result of European opposition (discussed in Despite the recent decline in planting of genetically engineered corn that occurred as a result of European opposition (discussed in chapter 8 chapter 8), some segments of the industry are doing very well.
One especially successful agricultural biotechnology company is Monsanto, which has played an unusually active-some might say aggressive-role in the industry. Monsanto is a multinational company based in St. Louis, Missouri, whose corporate motto used to be Food, Health, Hope Food, Health, Hope.8 After the company merged with Pharmacia & Upjohn in 2000 to form an agricultural unit of Pharmacia, it changed the slogan to After the company merged with Pharmacia & Upjohn in 2000 to form an agricultural unit of Pharmacia, it changed the slogan to A Single Focus: Agriculture/A Renewed Purpose: Value A Single Focus: Agriculture/A Renewed Purpose: Value. Monsanto employed about 14,000 people worldwide in 2002. Its agricultural biotechnology products exceed financial expectations. Its stock price rose by 75% in 1995 and by another 70% in 1996; at that time, company officials estimated that their products would earn $2 billion by the year 2000, $67 billion by 2005, and $20 billion by 2010. By 2000, sales exceeded $5 billion, well ahead of projections.9 Not all companies are this fortunate or skilled. In 1998, for example, just 8 out of 350 publicly traded food biotechnology companies were profitable.10 Business a.n.a.lysts attribute the typically poor performance to uneven management, corporate shortsightedness, and product failures. Most companies were slow to invest sufficient funds in research, as was the U.S. government. Investors are leery of regulatory hurdles and consumer opposition. Financial imperatives require food biotechnology companies to work on projects that are technically feasible and likely to repay the costs of investment in short order. Thus, they focus research efforts on "input traits" that will make crops easier and less expensive to grow through control of weeds, plant diseases, ripening, insects, or herbicide-resistance, or will make foods last longer on the shelf and cost less to process. If these characteristics benefit the public, they do so invisibly. Most of the financial rewards go to the companies that produce the seeds and chemicals. In some situations, farmers also benefit. Business a.n.a.lysts attribute the typically poor performance to uneven management, corporate shortsightedness, and product failures. Most companies were slow to invest sufficient funds in research, as was the U.S. government. Investors are leery of regulatory hurdles and consumer opposition. Financial imperatives require food biotechnology companies to work on projects that are technically feasible and likely to repay the costs of investment in short order. Thus, they focus research efforts on "input traits" that will make crops easier and less expensive to grow through control of weeds, plant diseases, ripening, insects, or herbicide-resistance, or will make foods last longer on the shelf and cost less to process. If these characteristics benefit the public, they do so invisibly. Most of the financial rewards go to the companies that produce the seeds and chemicals. In some situations, farmers also benefit.11 Monsanto applies its research budget for agricultural biotechnology, which exceeds the combined total of all the publicly funded tropical research inst.i.tutes in the world, almost exclusively to temperate-zone agricultural problems. The company brilliantly designs its princ.i.p.al agricultural products to establish control of the entire industry. Its flags.h.i.+p product is the herbicide Roundup. Monsanto scientists genetically engineer soybeans and corn to be "Roundup Ready," so their crops grow happily when doused with that herbicide while the competing weeds are killed. Farmers who buy Monsanto's seeds also buy Monsanto's herbicide. The company began selling Roundup Ready soybeans in 1996; just two years later, farmers planted them on one-third of U.S. soybean farmland, covering 25 million acres. The company's research "pipeline" mainly emphasizes Roundup Ready crops designed for animal feed. Monsanto's emphasis on these crops is understandable; annual sales of Roundup exceed those of the next six leading herbicides combined. The company also produces a variety of crops genetically engineered to contain a toxin derived from Bacillus thuringiensis Bacillus thuringiensis ( (Bt). As we saw in the introductory chapter, the Bt Bt toxin inhibits the growth of insect pests and has been used for years as a spray on organic farms. Monsanto's patent-protected innovation was to genetically engineer the toxin inhibits the growth of insect pests and has been used for years as a spray on organic farms. Monsanto's patent-protected innovation was to genetically engineer the Bt Bt toxin into the plant itself so that insect resistance would not wash off in the rain. toxin into the plant itself so that insect resistance would not wash off in the rain.
Monsanto's crops grow mainly in the United States and other industrialized countries. Because developing countries lack a viable market for such products, few agricultural biotechnology companies can afford to invest in solutions to the food problems of the developing world. The agricultural needs of developing countries are well defined, and numerous private and public agencies support useful projects, but these funding sources are not coordinated and often tend to favor the priorities of donors more than recipients.12 For years, Dr. Roger Beachy, the director of a U.S. biotechnology research inst.i.tute devoted to improving crops in developing countries, complained that he could get little support from industry beyond permission to use patent-protected techniques "for specific crops under certain circ.u.mstances." For years, Dr. Roger Beachy, the director of a U.S. biotechnology research inst.i.tute devoted to improving crops in developing countries, complained that he could get little support from industry beyond permission to use patent-protected techniques "for specific crops under certain circ.u.mstances."13 As complaints about the disparity between the promises and the realities of food biotechnology became more strident, companies began to put more resources into projects that might benefit the developing world. Monsanto's scientists, for example, are genetically engineering oilseeds to contain beta-carotene, a precursor of vitamin A. This vitamin is especially lacking in undernourished populations, and its addition to the diet produces an almost miraculous range of health improvements.14 Development of such products is time-consuming and expensive, and success is uncertain. Companies introduced genetically engineered papaya in Hawaii, for example, to replenish an entire industry ravaged by viral disease. The fruit grew well in the first seasons, but its developers remain cautious about its long-term viability: "We'd all be nuts to say that this is the final solution. . . . Biological systems evolve." Development of such products is time-consuming and expensive, and success is uncertain. Companies introduced genetically engineered papaya in Hawaii, for example, to replenish an entire industry ravaged by viral disease. The fruit grew well in the first seasons, but its developers remain cautious about its long-term viability: "We'd all be nuts to say that this is the final solution. . . . Biological systems evolve."15 This comment reflects yet another reality; it is one thing to develop a food in a laboratory but quite another to grow it successfully under field conditions. A 1994 statement by one business a.n.a.lyst still applies: "Nearly 20 years into the gene-splicing revolution . . . no one has cured cancer or produced a bioengineered miracle of loaves and fishes for a hungry third world. The industry is still peddling dreams." This comment reflects yet another reality; it is one thing to develop a food in a laboratory but quite another to grow it successfully under field conditions. A 1994 statement by one business a.n.a.lyst still applies: "Nearly 20 years into the gene-splicing revolution . . . no one has cured cancer or produced a bioengineered miracle of loaves and fishes for a hungry third world. The industry is still peddling dreams."16 Such doubts enrage industry supporters in the United States and, sometimes, in developing countries. Florence Wambugu, for example, is a plant pathologist from Kenya who has worked with Monsanto since 1992 to develop a genetically modified sweet potato that can survive infection from a virus that otherwise would greatly reduce crop yields. At the Tufts University conference I attended in 2001, she predicted that the bioengineered potato would increase worldwide sweet potato production by at least 15%, increase the income of farmers by $41 million, and improve the food security of 1 million people-without any increase in the costs of production. Ms. Wambugu is an eloquent and forceful promoter of biotechnology as the solution to worldwide food shortages, and she does not mince words about the harm caused by "antibiotech lobbies": Antibiotechnology protesters . . . deny developing countries like my home, Kenya, the resources to develop a technology that can help alleviate hunger, malnutrition and poverty. . . . As an African, I know that biotech is not a panacea. It cannot solve problems of inept or corrupt governments, underfunded research, unsound agricultural policy, or a lack of capital . . . but as a scientist, I also know that biotech is a powerful new tool that can help address some of the agricultural problems that plague Africa. The protesters have fanned the flames of mistrust of genetically modified foods through a campaign of misinformation. These people and organizations have become adept at playing on the media's appet.i.te for controversy to draw attention to their cause. But the real victim in this controversy is the truth. . . . I know of what I speak, because I grew up barefoot and hungry.17 In 2001, her sweet potato was in field trials, and the level of its productivity or acceptance would not be known for some time. Nevertheless, Monsanto has used the potato in its public relations campaigns since 1996 ("the sweet potato project will ultimately be a major contribution to food security for some of the poorest farmers in the world"), and the Biotechnology Information Council, which runs an industry-sponsored public relations campaign, also uses her work: "Florence Wambugu helped develop sweet potato varieties that are resistant to a complex set of viruses that can wipe out three-fourths of Kenyan farmers' harvest. . . . Similar techniques are being used to improve other staple crops of the developing world, including ca.s.sava, banana, and potato."18 These statements are promises. The crops are not yet in production, but the public relations materials do not emphasize that point. These statements are promises. The crops are not yet in production, but the public relations materials do not emphasize that point.
The most highly publicized example of the gap between promises and reality is "Golden Rice," genetically engineered to contain beta-carotene, a precursor of vitamin A. Although this rice also is not yet in production, it has been the industry's primary advertising tool to promote the humanitarian benefits of food biotechnology (see figure 12 figure 12). This rice raises a variety of issues that ill.u.s.trate some further points about the interweaving of science and politics in food biotechnology, as we will now see MAKING RICE "GOLDEN"
Much of the promise of food biotechnology depends on its science, but the realities depend on social as well as scientific factors. Nowhere is this distinction better ill.u.s.trated than in the case of Golden Rice. To understand why the interplay between the scientific and societal issues makes genetically modified foods so political political, we need to begin with an explanation of the extraordinary scientific achievement involved in creating Golden Rice.
Biotechnology versus Traditional Plant Genetics Scientists who are puzzled by public distrust of food biotechnology tend to see its techniques as extensions of those of traditional plant genetics but superior because they are more efficient and precise. Traditional plant breeding can be tedious. Suppose, for example, that you would like to create a tomato with a thicker skin so it can be transported without getting crushed. Using the typical genetic methods, you would grow many kinds of tomatoes and look for a rare plant that produces tomatoes with thicker skins. You might also treat tomato embryos with chemicals or radiation to induce mutations; if you are lucky, a mutation will lead to fruit with a thicker skin. You then grow seeds from these tomatoes into plants, select progeny plants with thicker skins, cross them (through pollination) with tomato plants with other desirable traits, and, eventually, end up with thick-skinned tomatoes that breed true. A process like this involves luck as well as skill, takes an average of six to eight years of growing cycles, and can (and often does) result in a tasteless supermarket tomato. Other such manipulations created the full array of fruits, vegetables, and crops that make our food supply so abundant. It is safe to say that virtually all plants that const.i.tute part of today's food supply were genetically manipulated in one way or another. Traditional genetic manipulations permit the transfer of genes only between members of the same species or those that are closely related-apples and pears, for example. In contrast, agricultural biotechnology extends these techniques to address problems of efficiency, time, and species limits on transferable traits.
[image]
FIGURE 12. This advertis.e.m.e.nt for the benefits of Golden Rice is part of an industry public relations campaign to promote public acceptance of genetically modified foods; it appeared frequently in 2001 in publications such as the New Yorker, Scientific American New Yorker, Scientific American, and the New York Times New York Times. The text fails to emphasize that the rice, which "could help alleviate more suffering and illness than any single medicine," is not yet available.
Because both traditional plant genetics and biotechnology involve similar manipulations and because they both achieve the same result-insertion of new segments of DNA into a plant's existing DNA-biotechnologists maintain that the plants they develop are no different from those produced in old-fas.h.i.+oned ways, and should not be viewed or treated differently by regulatory agencies or the public. As we will see (and as the appendix explains in further detail), the steps involved in creating a transgenic plant are numerous and complex, and they introduce DNA segments that may come from unrelated organisms. Do these differences matter? The response is no no if one focuses on the similarities: DNA is DNA no matter where it comes from. The response is if one focuses on the similarities: DNA is DNA no matter where it comes from. The response is yes yes if one focuses on differences or the societal implications of the technology. Points of view govern such responses and lead to political controversy. if one focuses on differences or the societal implications of the technology. Points of view govern such responses and lead to political controversy.
Golden Rice: The Science Plant bioengineering is accomplished through recombinant recombinant DNA technology, through which the DNA segments that comprise a desirable gene from bacteria, for example, are inserted (recombined) permanently into the DNA of an entirely different organism-in this case, a plant. Scientists using recombinant techniques have created insect- and herbicide-resistant crops by taking genes from bacteria and transferring them to corn and soybeans. To develop Golden Rice, they recombined genes and DNA regulatory segments from daffodils, peas, viruses, and bacteria to induce rice to make beta-carotene in its endosperm-the white, starchy part of the grain. Rice, like all grains, consists of three princ.i.p.al parts: a surrounding sheath of nutrient-rich bran, an inner endosperm containing starch and a little protein, and an embryo, which draws on the energy and nutrients in the grain when it begins to grow into a plant (see DNA technology, through which the DNA segments that comprise a desirable gene from bacteria, for example, are inserted (recombined) permanently into the DNA of an entirely different organism-in this case, a plant. Scientists using recombinant techniques have created insect- and herbicide-resistant crops by taking genes from bacteria and transferring them to corn and soybeans. To develop Golden Rice, they recombined genes and DNA regulatory segments from daffodils, peas, viruses, and bacteria to induce rice to make beta-carotene in its endosperm-the white, starchy part of the grain. Rice, like all grains, consists of three princ.i.p.al parts: a surrounding sheath of nutrient-rich bran, an inner endosperm containing starch and a little protein, and an embryo, which draws on the energy and nutrients in the grain when it begins to grow into a plant (see figure 13 figure 13). Rice makes small amounts of beta-carotene in its bran layers, but not in the endosperm. Most people just eat the endosperm, however, because millers remove the bran layers when they convert brown rice to white rice (which is why white rice in the United States is enriched with several vitamins and iron).
[image]
FIGURE 13. The metabolic steps through which plants make beta-carotene from precursor molecules, and animals convert beta-carotene to vitamin A. An enzyme carries out each step. Rice bran contains information for the complete set of enzymes to make beta-carotene, but some enzymes are inactive in the endosperm. To create Golden Rice, scientists obtain genes (DNA) for the missing enzymes from other plants and bacteria and insert them into the DNA of rice (see tables 12 tables 12 and and 16 16, pages 158 pages 158 and and 280 280).
Rice bran and highly pigmented fruits and vegetables (such as melons or carrots) make beta-carotene in a series of steps in which precursor molecules are converted to beta-carotene by specific enzymes (which are proteins), one for each step. Rice endosperm lacks three of the required enzymes. To insert beta-carotene, researchers Ingo Potrykus and Peter Beyer and their colleagues in Switzerland and Germany obtained genes for the missing enzymes from daffodils and bacteria. They also isolated genes or regulatory DNA segments from peas, viruses, and other bacteria to help the recombinant enzymes function in rice endosperm. After a decade of effort during the 1990s, the techniques worked. The scientists made rice that contained beta-carotene and identified it immediately by its yellow color (hence: Golden Rice). They published this work in 2000. Figure 13 Figure 13 ill.u.s.trates the pathway of biosynthesis of beta-carotene and the enzymes replaced by genetic engineering. The pathway ill.u.s.trates an important distinction: beta-carotene is not the same as vitamin A but is a ill.u.s.trates the pathway of biosynthesis of beta-carotene and the enzymes replaced by genetic engineering. The pathway ill.u.s.trates an important distinction: beta-carotene is not the same as vitamin A but is a precursor precursor of the actual vitamin. We (and other mammals) have enzymes that convert beta-carotene to vitamin A in our bodies. of the actual vitamin. We (and other mammals) have enzymes that convert beta-carotene to vitamin A in our bodies.19 The technical challenges involved in moving genes from one organism to another-daffodils and bacteria to rice, for example-are daunting, even to experts. Scientists must find the genes for the missing enzymes, reproduce them, and make them function. The "make function" part is particularly challenging. Genes do not work independently. They have to be regulated regulated, which means in this case that the rice needs to be "told" when, where, and for how long the genes for making beta-carotene should do so. Scientists must also find, duplicate, and transfer the genes or DNA segments for these regulatory functions into the rice along with the genes for the missing enzymes. Accomplis.h.i.+ng these tasks is a technical tour de force tour de force-an art as well as a science-not least because of the extraordinary number of genes and factors required, each requiring its own separate bioengineering step carried out in just the right order. As an ill.u.s.tration of the complexity of this work, table 17 table 17 in the appendix ( in the appendix (page 302) summarizes the less less complicated of the two approaches used to insert beta-carotene genes into rice. complicated of the two approaches used to insert beta-carotene genes into rice.
Complicated as they are, the genetic engineering steps are only the first first part of realizing the humanitarian benefits of Golden Rice. The inserted genes must be transmitted to seeds; the rice must continue to make beta-carotene when taken out of the laboratory and grown in fields; people must accept, buy, and eat the rice; and the beta-carotene must be absorbed, split into vitamin A, and function in the human body. part of realizing the humanitarian benefits of Golden Rice. The inserted genes must be transmitted to seeds; the rice must continue to make beta-carotene when taken out of the laboratory and grown in fields; people must accept, buy, and eat the rice; and the beta-carotene must be absorbed, split into vitamin A, and function in the human body. Table 12 Table 12 lists these requirements in greater detail. These additional tasks also can be difficult to accomplish. One production problem, for example, is the relative instability of transgenic plants with multiple inserted genes; such plants tend to lose the transgenic traits over several generations. Another is that the scientists engineered beta-carotene into a variety of rice that grows best in temperate zones. To succeed in developing countries, the technology must be transferred to locally grown varieties. lists these requirements in greater detail. These additional tasks also can be difficult to accomplish. One production problem, for example, is the relative instability of transgenic plants with multiple inserted genes; such plants tend to lose the transgenic traits over several generations. Another is that the scientists engineered beta-carotene into a variety of rice that grows best in temperate zones. To succeed in developing countries, the technology must be transferred to locally grown varieties.
TABLE 12. Research steps required to genetically engineer and to produce and use Golden Rice containing beta-carotene, a precursor of vitamin A Research steps required to genetically engineer and to produce and use Golden Rice containing beta-carotene, a precursor of vitamin A Basic Research (see (see table 17 table 17 in appendix for further details) in appendix for further details) Isolate the desired genes and regulatory DNA segments from daffodils, bacteria, peas, and viruses.Transfer the genes and segments to rice embryos.Grow the embryos; select the rare embryos that accept the desired genes and segments.Grow the transgenic embryos into plants.Harvest seeds from the plants.Test the seeds for beta-carotene.Repeat the procedures in rice strains able to grow in tropical climates.
Production Research Grow the transgenic rice for several generations to ensure the stability of the beta-carotene trait.Evaluate the plants for environmental effects, presence of allergens, changes in nutrient composition, or unwanted effects on yield.Obtain regulatory approval to grow the rice commercially.Obtain regulatory approval to market the rice.Produce the rice in sufficient quant.i.ties for distribution and marketing.
Consumer Research Conduct studies to determine the degree of consumer acceptance of the rice.Conduct dietary studies to evaluate patterns of consumption of the rice among vitamin Adeficient individuals and population groups.
Clinical Research Conduct biochemical studies to determine how much beta-carotene is absorbed from the rice, and whether consuming the rice increases levels of vitamin A in the body.Conduct clinical studies to determine whether consuming the rice is a.s.sociated with a reduction in symptoms of vitamin A deficiency and improvements in health and survival among individuals and population groups.
The degree of acceptance by consumers is also a matter of concern. Preliminary surveys suggested that some people found the yellow color unattractive; they thought someone might have urinated on the rice. Scientists can remove the undesirable color by inserting the genes for the additional enzymes in the pathway to vitamin A (which is colorless), but these steps only add to the technical difficulties. Table 12 Table 12 explains why promotion of Golden Rice as a means to prevent vitamin A deficiency is premature. At best several more years of work will be needed to bring it to market. explains why promotion of Golden Rice as a means to prevent vitamin A deficiency is premature. At best several more years of work will be needed to bring it to market.
Golden Rice: The Politics White rice is the princ.i.p.al source of energy (calories) for one-third or more of the world's population, but it is not a source of vitamin A. Only animals make vitamin A; plants make beta-carotene, its precursor. The lack of vitamin A is the single most important cause of blindness among children in developing countries and a major contributor to deaths among malnourished children and adults. Children who are even mildly deficient in vitamin A are at increased risk for early death, but health authorities can prevent an astonis.h.i.+ng proportion of such deaths-more than half-with supplements of vitamin A (not beta-carotene). Supplements are relatively inexpensive and need to be taken once every six months or so, but because they cannot always be obtained by the people who need them most, fortification of a commonly consumed food might be another way to solve a serious world health problem. beta-carotene). Supplements are relatively inexpensive and need to be taken once every six months or so, but because they cannot always be obtained by the people who need them most, fortification of a commonly consumed food might be another way to solve a serious world health problem.20 Since 1984, the Rockefeller Foundation has dispensed about $4 million annually to fund genetics projects to improve one characteristic or another of rice plants, and it considers Golden Rice to be the greatest achievement of this program. Moving Golden Rice beyond the research stage, however, unexpectedly encountered political problems. Ironically, one of the difficulties was a confrontation with patent rights, as a "thicket of intellectual property claims" governed use of the technology. The companies most likely to benefit from the public relations generated by Golden Rice, among them Monsanto and AstraZeneca, hold proprietary patent rights to as many as 70 of the materials or DNA segments needed for its construction. To solve the legal problems connected with using the technology, Dr. Potrykus and his colleagues contracted with AstraZeneca to market the rice in the United States and other industrial markets. In return, AstraZeneca agreed to help make the technology available to the developing world. It gave the technology to the International Rice Research Inst.i.tute in the Philippines where scientists are crossing Golden Rice with locally grown varieties. AstraZeneca also said it would give the Golden Rice seeds to farmers earning less than $10,000 a year (a figure that includes most farmers in developing countries) and allow farmers to save the seeds to plant in future years. Monsanto also agreed to give up its intellectual property rights for this rice.21 These concessions appear exceedingly generous, but Golden Rice is unlikely to have much commercial potential in developing countries. Its public relations value, however, is enormous. In July 2000, the cover of Time Time displayed a photograph of Dr. Potrykus with the headline "This rice could save a million kids a year . . . but protesters believe such genetically modified foods are bad for us and our planet. Here's why." The story noted that it was "no wonder the biotech industry sees Golden Rice as a powerful ally in its struggle to win public acceptance. No wonder its critics see it as a cynical ploy." displayed a photograph of Dr. Potrykus with the headline "This rice could save a million kids a year . . . but protesters believe such genetically modified foods are bad for us and our planet. Here's why." The story noted that it was "no wonder the biotech industry sees Golden Rice as a powerful ally in its struggle to win public acceptance. No wonder its critics see it as a cynical ploy."22 Cynics might indeed raise eyebrows at the advertis.e.m.e.nt shown in Cynics might indeed raise eyebrows at the advertis.e.m.e.nt shown in figure 14 figure 14, a component of the biotechnology industry's public relations campaign in 2001. The advertis.e.m.e.nt features a photograph of a child of indeterminate ethnicity eating a "vitamin-enriched" breakfast cereal presumably made from Golden Rice. It says, "Thanks to biotechnology, researchers are developing a new kind of rice with beta-carotene. . . . In the future it could help prevent serious illnesses, such as blindness or anemia, for many people in developing parts of the world."
Dr. Potrykus-frustrated by the enc.u.mbrances of industry patent rights on the one hand and objections by antibiotechnology advocates on the other-emphasizes the humanitarian benefits of his research. He told the Tufts University conference that the 40,000 people dying from malnutrition every day need the technology just to survive. Malnutrition, he said, pose[s] immense medical problems for developing countries. Traditional interventions are helpful, but require additional and complementary actions. . . . Applied in "humanitarian projects" they could substantially and sustainably improve the health and life of the poor. Whether the poor will benefit, does neither depend upon scientific, patent right, or economic problems, nor upon socioeconomic, consumer health, or environmental risks. It depends mainly upon the political "success" of radical anti-GMO organizations. Those who try to prevent careful exploitation in humanitarian projects must be taken responsible for their damage.23 By "those," Dr. Potrykus meant Greenpeace: "Is there any problem left that could interfere with the exploitation of 'Golden Rice' to the benefit of the poor and disadvantaged in developing countries? It is unfortunate that the answer is yes: Greenpeace . . . and a.s.sociated GMO opponents regard 'Golden Rice' as a 'Trojan Horse.'. . . By their singular logic, the success of 'Golden Rice' has to be prevented under all circ.u.mstances, irrespective of the damage to those for whose interest Greenpeace pretends to act."24 Dr. Potrykus is correct in his a.s.sessment of the motivations of Greenpeace. From that organization's standpoint, Golden Rice obscures fundamental issues of societal values-in this case, poverty and control over resources-and is a techno-fix imposed by corporations and scientists without consulting recipients about whether they want it or not. Greenpeace says that the true purpose of Golden Rice is to convince people to accept genetically modified foods. Dr. Potrykus is correct in his a.s.sessment of the motivations of Greenpeace. From that organization's standpoint, Golden Rice obscures fundamental issues of societal values-in this case, poverty and control over resources-and is a techno-fix imposed by corporations and scientists without consulting recipients about whether they want it or not. Greenpeace says that the true purpose of Golden Rice is to convince people to accept genetically modified foods.
[image]
FIGURE 14. This biotechnology industry advertis.e.m.e.nt appeared late in 2001 inside the front cover of Food Safety Food Safety, a publication of the National Restaurant a.s.sociation's Educational Foundation. The text suggests that Golden Rice could help prevent nutritional deficiencies among people in the developing world, presumably by replacing the current vitamin-enriched breakfast cereals.
If Greenpeace frustrates scientists and biotechnology industry officials, it is in part because its tactics are so effective. For one thing, Greenpeace fights science with science. In February 2001, the group challenged the fundamental premise (and promise) of Golden Rice. Greenpeace calculated that adults would have to eat at least 20 pounds pounds (9 kilograms) of Golden Rice to meet daily vitamin A recommendations. Greenpeace called Golden Rice nothing but "fool's gold" and said, "It is shameful that the biotech industry is using starving children to promote a dubious product. . . . This isn't about solving childhood blindness, it's about solving biotech's public relations problem." (9 kilograms) of Golden Rice to meet daily vitamin A recommendations. Greenpeace called Golden Rice nothing but "fool's gold" and said, "It is shameful that the biotech industry is using starving children to promote a dubious product. . . . This isn't about solving childhood blindness, it's about solving biotech's public relations problem."25 Greenpeace did its homework. It took at face value the scientists' own estimate that a daily intake of 300 grams (nearly 11 ounces) of Golden Rice should provide the equivalent equivalent of 100 units of vitamin A. As noted earlier, beta-carotene must be converted to vitamin A in the body. This process is usually incomplete, however, and the amount that is converted into vitamin A is a matter of sharp debate. The scientists who developed Golden Rice a.s.sumed that 6 molecules of beta-carotene would yield 1 of vitamin A, whereas U.S. estimates suggest a conversion ratio of 12 to 1. of 100 units of vitamin A. As noted earlier, beta-carotene must be converted to vitamin A in the body. This process is usually incomplete, however, and the amount that is converted into vitamin A is a matter of sharp debate. The scientists who developed Golden Rice a.s.sumed that 6 molecules of beta-carotene would yield 1 of vitamin A, whereas U.S. estimates suggest a conversion ratio of 12 to 1.
Greenpeace took the scientists' figures and compared them to recommended levels of vitamin A intake for the U.S. population. By U.S. standards, 300 grams (11 ounces) of Golden Rice provides one-third the recommended level of daily intake of vitamin A for a child aged one to three years, one-seventh the level recommended for an adult woman, and one-ninth the level for an adult man. By such standards, young children would need to eat nearly 33 ounces of raw rice per day, which, when cooked, would amount to 99 ounces, or about 6 pounds-an absurdly large amount. If the Golden Rice scientists had used the higher U.S. conversion ratio, that quant.i.ty doubles to an even more absurd 12 pounds.26 It must be understood that the U.S. standard is deliberately set high to meet the nutritional needs of about 98% of the population; people with average requirements can prevent vitamin A deficiency at much lower levels of intake.27 Nevertheless, to meet just 10% of the U.S. standard, young children would still need to eat more than a pound of cooked rice a day. The Greenpeace a.n.a.lysis made it clear that on quant.i.tative grounds alone, Golden Rice would const.i.tute-at best-a partial solution to health problems caused by vitamin A deficiency. Nevertheless, to meet just 10% of the U.S. standard, young children would still need to eat more than a pound of cooked rice a day. The Greenpeace a.n.a.lysis made it clear that on quant.i.tative grounds alone, Golden Rice would const.i.tute-at best-a partial solution to health problems caused by vitamin A deficiency.
As might be antic.i.p.ated, the Greenpeace estimations elicited outraged arguments from scientists and the industry. As a nutritionist, I particularly appreciated the arguments because they raged around the kinds of basic questions my colleagues and I like to discuss in nutrition science courses: What standards are appropriate for the intake of nutrients by individuals and populations? How much beta-carotene is converted to vitamin A in the body? How much vitamin A is required to prevent or alleviate the symptoms or consequences of deficiency? The arguments also dealt with an important question in applied applied nutrition: Should nutritional standards for developing countries be the same as or lower than those for industrialized countries? This question is political rather than scientific because of its implications: lower nutrient standards make populations appear to be better nourished. They also make Golden Rice appear to be more effective. n
Safe Food: Bacteria, Biotechnology, And Bioterrorism Part 3
You're reading novel Safe Food: Bacteria, Biotechnology, And Bioterrorism Part 3 online at LightNovelFree.com. You can use the follow function to bookmark your favorite novel ( Only for registered users ). If you find any errors ( broken links, can't load photos, etc.. ), Please let us know so we can fix it as soon as possible. And when you start a conversation or debate about a certain topic with other people, please do not offend them just because you don't like their opinions.
Safe Food: Bacteria, Biotechnology, And Bioterrorism Part 3 summary
You're reading Safe Food: Bacteria, Biotechnology, And Bioterrorism Part 3. This novel has been translated by Updating. Author: Marion Nestle already has 619 views.
It's great if you read and follow any novel on our website. We promise you that we'll bring you the latest, hottest novel everyday and FREE.
LightNovelFree.com is a most smartest website for reading novel online, it can automatic resize images to fit your pc screen, even on your mobile. Experience now by using your smartphone and access to LightNovelFree.com
- Related chapter:
- Safe Food: Bacteria, Biotechnology, And Bioterrorism Part 2
- Safe Food: Bacteria, Biotechnology, And Bioterrorism Part 4
RECENTLY UPDATED NOVEL

Complete Martial Arts Attributes
Complete Martial Arts Attributes Chapter 2925: Blood Sea Domain! Huge Enlightenment! Transformation! (3) View : 2,878,075
Cultivating In Secret Beside A Demoness
Cultivating In Secret Beside A Demoness Chapter 1314: A Glimpse of the Great Dao (1) View : 505,184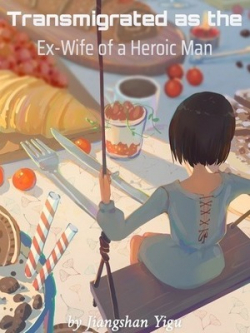
Transmigrated as the Ex-Wife of a Heroic Man
Transmigrated as the Ex-Wife of a Heroic Man Chapter 1309: He Does Not Belong to Me View : 576,960
My Girlfriend is a Zombie
My Girlfriend is a Zombie Chapter 842: Unexpected Contact View : 2,300,817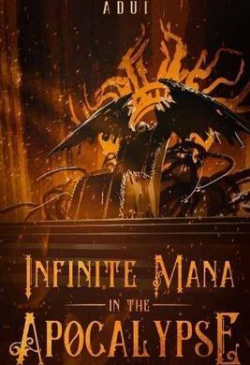
Infinite Mana In The Apocalypse
Infinite Mana In The Apocalypse Chapter 3448 A Spark! II View : 4,818,660
Lord of Mysteries 2: Circle of Inevitability
Lord of Mysteries 2: Circle of Inevitability Chapter 1162: A Distant Planet View : 664,871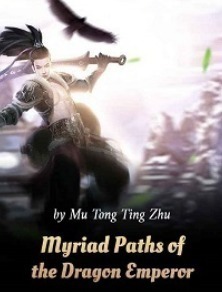
Myriad Paths of the Dragon Emperor
Myriad Paths of the Dragon Emperor Chapter 3031-bullying View : 1,581,088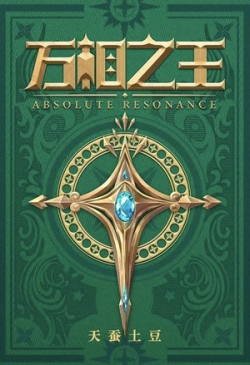