Death By Black Hole Part 7
You’re reading novel Death By Black Hole Part 7 online at LightNovelFree.com. Please use the follow button to get notification about the latest chapter next time when you visit LightNovelFree.com. Use F11 button to read novel in full-screen(PC only). Drop by anytime you want to read free – fast – latest novel. It’s great if you could leave a comment, share your opinion about the new chapters, new novel with others on the internet. We’ll do our best to bring you the finest, latest novel everyday. Enjoy!
For the map of the famous cosmic microwave background, some areas are hotter than average. And, as must be the case, some areas are cooler than average. The range spans about one one-hundred-thousandth of a degree. How do you display this fact? Make the hot spots blue, and the cold spots red, or vice versa. In either case, a very small fluctuation in temperature shows up as an obvious difference on the picture.
Sometimes the public sees a full-color image of a cosmic object that was photographed using invisible light such as infrared, or radio waves. In most of these cases, we have a.s.signed three colors, usually red, green, and blue (or "RGB" for short) to three different regions within the band. From this exercise, a full-color image can be constructed as though we were born with the capacity to see colors in these otherwise invisible parts of the spectrum.
The lesson is that common colors in common parlance can mean very different things to scientists than they do to everybody else. For the occasions when astrophysicists choose to speak unambiguously, we do have tools and methods that quantify the exact color emitted or reflected by an object, avoiding the tastes of the image maker or the messy business of human color perception. But these methods are not public-friendly. They involve the logarithmic ratio of the flux emitted by an object as measured through multiple filters in a well-defined system corrected for the detector's sensitivity profile. (See, I told you it wasn't public-friendly.) When that ratio decreases, for example, the object is technically turning blue no matter what color it appears to be.
THE VAGARIES OF human color perception took their toll on the wealthy American astronomer and Mars fanatic Percival Lowell. During the late 1800s and early 1900s, he made quite detailed drawings of the Martian surface. To make such observations, you need steady dry air, which reduces smearing of the planet's light en route to your eyeball. In the arid air of Arizona, atop Mars Hill, Lowell founded the Lowell Observatory in 1894. The iron-rich, rusty surface of Mars looks red at any magnification, but Lowell also recorded many patches of green at the intersections of what he described and ill.u.s.trated as ca.n.a.ls-artificial waterways, presumably made by real live Martians who were eager to distribute precious water from the polar icecaps to their cities, hamlets, and surrounding farmlands. human color perception took their toll on the wealthy American astronomer and Mars fanatic Percival Lowell. During the late 1800s and early 1900s, he made quite detailed drawings of the Martian surface. To make such observations, you need steady dry air, which reduces smearing of the planet's light en route to your eyeball. In the arid air of Arizona, atop Mars Hill, Lowell founded the Lowell Observatory in 1894. The iron-rich, rusty surface of Mars looks red at any magnification, but Lowell also recorded many patches of green at the intersections of what he described and ill.u.s.trated as ca.n.a.ls-artificial waterways, presumably made by real live Martians who were eager to distribute precious water from the polar icecaps to their cities, hamlets, and surrounding farmlands.
Let's not worry here about Lowell's alien voyeurism. Instead, let's just focus on his ca.n.a.ls and green patches of vegetation. Percival was the unwitting victim of two well-known optical illusions. First, in almost all circ.u.mstances, the brain attempts to create visual order where there is no order at all. The constellations in the sky are prime examples-the result of imaginative, sleepy people a.s.serting order on a random a.s.sortment of stars. Likewise, Lowell's brain interpreted uncorrelated surface and atmospheric features on Mars as large-scale patterns.
The second illusion is that gray, when viewed next to yellow-red, appears green-blue, an effect first pointed out by the French chemist M. E. Chevreul in 1839. Mars displays a dull red on its surface with regions of gray-brown. The green-blue arises from a physiological effect in which a color-neutral area surrounded by a yellow-orange appears bluish green to the eye.
In another peculiar but less embarra.s.sing physiological effect, your brain tends to color balance the lighting environment in which you are immersed. Under the canopy of a rain forest, for example, where nearly all of the light that reaches the jungle floor has been filtered green (for having pa.s.sed through leaves), a milk-white sheet of paper ought to look green. But it doesn't. Your brain makes it white in spite of the lighting conditions.
In a more common example, walk past a window at night while the people inside are watching television. If the TV is the only light in the room, the walls will glow a soft blue. But the brains of the people immersed in the light of the television actively color balance their walls and see no such discoloration around them. This bit of physiological compensation may prevent residents of our first Martian colony from taking notice of the prevailing red of their landscape. Indeed, the first images sent back to Earth in 1976 from the Viking Viking lander, though pale, were purposefully color-tinted to a deep red so that they would fulfill the visual expectations of the press. lander, though pale, were purposefully color-tinted to a deep red so that they would fulfill the visual expectations of the press.
AT MID-TWENTIETH CENTURY, the night sky was systematically photographed from a location just outside San Diego, California. This seminal database, known as the Palomar Observatory Sky Survey, served as the foundation for targeted, follow-up observations of the cosmos for an entire generation. The cosmic surveyors photographed the sky twice, using identical exposures in two different kinds of black-and-white Kodak film-one ultrasensitive to blue light, the other ultrasensitive to red. (Indeed the Kodak corporation had an entire division whose job it was to serve the photographic frontier of astronomers, whose collective needs helped to push Kodak's R&D to its limits.) If a celestial object piqued your interest, you'd be sure to look at both the red-and blue-sensitive images as a first indication of the quality of light it emits. For example, extremely red objects are bright on the red image but barely visible on the blue. This kind of information informed subsequent observing programs for the targeted object.
Although modestly sized compared with the largest ground-based telescopes, the 94-inch Hubble s.p.a.ce Telescope Hubble s.p.a.ce Telescope has taken spectacular color images of the cosmos. The most memorable of these photographs are part of the Hubble Heritage series that will secure the telescope's legacy in the hearts and minds of the public. What astrophysicists do to make color images will surprise most people. First, we use the same digital CCD technology found in household camcorders, except that we used it a decade before you did and our detectors are much, much higher quality. Second, we filter the light in any one of several dozen ways before it hits the CCD. For an ordinary color photo, we obtain three successive images of the object, seen through broadband red, green, and blue filters. In spite of their names, taken together these filters span the entire visible spectrum. Next, we combine the three images in software the way the wetware of your brain combines the signals from the red-, green-, and blue-sensitive cones in your retina. This generates a color picture that greatly resembles what you would see if the iris in your eyeball were 94 inches in diameter. has taken spectacular color images of the cosmos. The most memorable of these photographs are part of the Hubble Heritage series that will secure the telescope's legacy in the hearts and minds of the public. What astrophysicists do to make color images will surprise most people. First, we use the same digital CCD technology found in household camcorders, except that we used it a decade before you did and our detectors are much, much higher quality. Second, we filter the light in any one of several dozen ways before it hits the CCD. For an ordinary color photo, we obtain three successive images of the object, seen through broadband red, green, and blue filters. In spite of their names, taken together these filters span the entire visible spectrum. Next, we combine the three images in software the way the wetware of your brain combines the signals from the red-, green-, and blue-sensitive cones in your retina. This generates a color picture that greatly resembles what you would see if the iris in your eyeball were 94 inches in diameter.
Suppose, however, that the object were emitting light strongly at specific wavelengths due to the quantum properties of its atoms and molecules. If we know this in advance, and use filters tuned to these emissions, we can narrow our image sensitivity to just these wavelengths, instead of using broadband RGB. The result? Sharp features pop out of the picture, revealing structure and texture that would otherwise go unnoticed. A good example lives in our cosmic backyard. I confess to having never actually seen Jupiter's red spot through a telescope. While sometimes it's paler than at other times, the best way to see it is through a filter that isolates the red wavelengths of light coming from the molecules in the gas clouds.
In the galaxy, oxygen emits a pure green color when found near regions of star formation, amid the rarefied gas of the interstellar medium. (This was the mysterious element "nebulium" described earlier.) Filter for it and oxygen's signature arrives at the detector unpolluted by any ambient green light that may also occupy the scene. The vivid greens that jump out of many Hubble Hubble images come directly from oxygen's nighttime emissions. Filter for other atomic or molecular species and the color images become a chemical probe of the cosmos. The images come directly from oxygen's nighttime emissions. Filter for other atomic or molecular species and the color images become a chemical probe of the cosmos. The Hubble Hubble can do this so well that it's gallery of famous color images bears little resemblance to cla.s.sical RGB images of the same objects taken by others who have tried to simulate the color response of the human eye. can do this so well that it's gallery of famous color images bears little resemblance to cla.s.sical RGB images of the same objects taken by others who have tried to simulate the color response of the human eye.
The debate rages over whether or not these Hubble Hubble images contain "true" colors. One thing is certain, they do not contain "false" colors. They are the actual colors emitted by actual astrophysical objects and phenomena. Purists insist that we are doing a disservice to the public by not showing cosmic colors as the human eye would perceive them. I maintain, however, that if your retina were tunable to narrow-band light, then you would see just what the images contain "true" colors. One thing is certain, they do not contain "false" colors. They are the actual colors emitted by actual astrophysical objects and phenomena. Purists insist that we are doing a disservice to the public by not showing cosmic colors as the human eye would perceive them. I maintain, however, that if your retina were tunable to narrow-band light, then you would see just what the Hubble Hubble sees. I further maintain that my "if" in the previous sentence is no more contrived than the "if" in "If your eyes were the size of large telescopes." sees. I further maintain that my "if" in the previous sentence is no more contrived than the "if" in "If your eyes were the size of large telescopes."
The question remains, if you added together the visible light of all light-emitting objects in the universe, what color would you get? In simpler phrasing, What color is the universe? Fortunately, some people with nothing better to do have actually calculated the answer to this question. After an erroneous report that the universe is a cross between medium aquamarine and pale turquoise, Karl Glazebrook and Ivan Baldry of Johns Hopkins University corrected their calculations and determined that the universe is really a light shade of beige, or perhaps, cosmic latte. Glazebrook and Baldry's chromatic revelations came from a survey of the visible light from more than 200,000 galaxies, occupying a large and representative volume of the universe.
The nineteenth-century English astronomer Sir John Herschel invented color photography. To the frequent confusion but occasional delight of the public, astrophysicists have been messing with the process ever sinceand will continue forever to do so.
EIGHTEEN.
COSMIC PLASMA.
Only in a few cases does a medical doctor's vocabulary overlap with that of the astrophysicist. The human skull has two "orbits" that shape the round cavities where our two eyeb.a.l.l.s go; your "solar" plexus sits in the middle of your chest; and our eyes, of course, each have "lenses"; but our body has no quasars and no galaxies in it. For orbits and lenses, the medical and astrophysical usage resemble each other greatly. The term "plasma," however, is common to both disciplines, yet the two meanings have nothing whatever to do with each other. A transfusion of blood plasma can save your life, but a brief encounter with a glowing blob of million-degree astrophysical plasma would leave a puff of smoke where you had just been standing.
Astrophysical plasmas are remarkable for their ubiquity, yet they're hardly ever discussed in introductory textbooks or the popular press. In popular writings, plasmas are often called the fourth state of matter because of a panoply of properties that set them apart from familiar solids, liquids, and gases. A plasma has freely moving atoms and molecules, just like a gas, but a plasma can conduct electricity as well as lock onto magnetic fields that pa.s.s through it. Most atoms within a plasma have had electrons stripped from them by one mechanism or another. And the combination of high temperature and low density is such that the electrons only occasionally recombine with their host atoms. Taken as a whole, the plasma remains electrically neutral because the total number of (negatively charged) electrons equals the total number of (positively charged) protons. But inside, plasma seethes with electrical currents and magnetic fields and so, in many ways, behaves nothing like the ideal gas we all learned about in high-school chemistry cla.s.s.
THE EFFECTS OF electric and magnetic fields on matter almost always dwarf the effects of gravity. The electrical force of attraction between a proton and an electron is forty powers of 10 stronger than their gravitational attraction. So strong are electromagnetic forces that a child's magnet easily lifts a paper clip off a tabletop in spite of Earth's formidable gravitational tug. Want a more interesting example? If you managed to extricate all the electrons from a cubic millimeter of atoms in the nose of the s.p.a.ce shuttle, and if you affixed them all to the base of the launchpad, then the attractive force would inhibit the launch. All engines would fire and the shuttle wouldn't budge. And if the Apollo astronauts had brought back to Earth all electrons from a thimbleful of lunar dust (while leaving behind on the Moon the atoms from which they came), then their force of attraction would exceed the gravitational attraction between Earth and the Moon in its...o...b..t. electric and magnetic fields on matter almost always dwarf the effects of gravity. The electrical force of attraction between a proton and an electron is forty powers of 10 stronger than their gravitational attraction. So strong are electromagnetic forces that a child's magnet easily lifts a paper clip off a tabletop in spite of Earth's formidable gravitational tug. Want a more interesting example? If you managed to extricate all the electrons from a cubic millimeter of atoms in the nose of the s.p.a.ce shuttle, and if you affixed them all to the base of the launchpad, then the attractive force would inhibit the launch. All engines would fire and the shuttle wouldn't budge. And if the Apollo astronauts had brought back to Earth all electrons from a thimbleful of lunar dust (while leaving behind on the Moon the atoms from which they came), then their force of attraction would exceed the gravitational attraction between Earth and the Moon in its...o...b..t.
The most conspicuous plasmas on Earth are fire, lightning, the trail of a shooting star, and of course, the electric shock you get after you shuffle around on your living room carpet in your wool socks and then touch a doork.n.o.b. Electrical discharges are jagged columns of electrons that abruptly move through the air when too many of them collect in one place. Across all the world's thunderstorms, Earth gets struck by lightning thousands of times per hour. The centimeter-wide air column through which a bolt of lightning travels becomes plasma in a fraction of a second as it is rendered aglow, having been raised to millions of degrees by these flowing electrons.
Every shooting star is a tiny particle of interplanetary debris moving so fast that it burns up in the air, harmlessly descending to Earth as cosmic dust. Almost the same thing happens to s.p.a.cecraft that reenter the atmosphere. Since their occupants don't want to land at their orbital speed of 18,000 miles per hour (about five miles per second), the kinetic energy must go somewhere. It turns into heat on the leading edge of the craft during reentry and is rapidly whisked away by the heat s.h.i.+elds. In this way, unlike shooting stars, the astronauts do not descend to Earth as dust. For several minutes during the descent, the heat is so intense that every molecule surrounding the s.p.a.ce capsule becomes ionized, cloaking the astronauts in a temporary plasma barrier, through which none of our communication signals can penetrate. This is the famous blackout period when the craft is aglow and Mission Control knows nothing of the astronauts' well-being. As the craft continues to slow down through the atmosphere, the temperature cools, the air gets denser, and the plasma state can no longer be sustained. The electrons go back home to their atoms and communications are quickly restored.
WHILE RELATIVELY RARE on Earth, plasmas comprise more than 99.99 percent of all the visible matter in the cosmos. This tally includes all stars and gas clouds that are aglow. Nearly all of the beautiful photographs taken by the on Earth, plasmas comprise more than 99.99 percent of all the visible matter in the cosmos. This tally includes all stars and gas clouds that are aglow. Nearly all of the beautiful photographs taken by the Hubble s.p.a.ce Telescope Hubble s.p.a.ce Telescope of nebulae in our galaxy depict colorful gas clouds in the form of plasma. For some, their shape and density are strongly influenced by the presence of magnetic fields from nearby sources. The plasma can lock a magnetic field into place and torque or otherwise shape the field to its whims. This marriage of plasma and magnetic field is a major feature of the Sun's 11-year cycle of activity. The gas near the Sun's equator rotates slightly faster than the gas near its poles. This differential is bad news for the Sun's complexion. With the Sun's magnetic field locked into its plasma, the field gets stretched and twisted. Sunspots, flares, prominences, and other solar blemishes come and go as the gnarly magnetic field punches through the Sun's surface, carrying solar plasma along with it. of nebulae in our galaxy depict colorful gas clouds in the form of plasma. For some, their shape and density are strongly influenced by the presence of magnetic fields from nearby sources. The plasma can lock a magnetic field into place and torque or otherwise shape the field to its whims. This marriage of plasma and magnetic field is a major feature of the Sun's 11-year cycle of activity. The gas near the Sun's equator rotates slightly faster than the gas near its poles. This differential is bad news for the Sun's complexion. With the Sun's magnetic field locked into its plasma, the field gets stretched and twisted. Sunspots, flares, prominences, and other solar blemishes come and go as the gnarly magnetic field punches through the Sun's surface, carrying solar plasma along with it.
Because of all the entanglement, the Sun flings up to a million tons per second of charged particles into s.p.a.ce, including electrons, protons, and bare helium nuclei. This particle stream-sometimes a gale and sometimes a zephyr-is more commonly known as the solar wind. This most famous of plasmas is responsible for ensuring that comet tails point away from the Sun, no matter if the comet is coming or going. By colliding with molecules in Earth's atmosphere near our magnetic poles, the solar wind is also the direct cause of auroras (the northern and southern lights), not only on Earth but on all planets with atmospheres and strong magnetic fields. Depending on a plasma's temperature and its mix of atomic or molecular species, some free electrons will recombine with needy atoms and cascade down the myriad energy levels within. En route, the electrons emit light of prescribed wavelengths. Auroras owe their beautiful colors to these electron hijinks, as do neon tubes, fluorescent lights, as well as those glowing plasma spheres offered for sale next to the lava lamps in tacky gift shops.
These days, satellite observatories give us an unprecedented capacity to monitor the Sun and report on the solar wind as though it were part of the day's weather forecast. My first-ever televised interview for the evening news was triggered by the report of a plasma pie hurled by the Sun directly at Earth. Everybody (or at least the reporters) was scared that bad things would happen to civilization when it hit. I told the viewers not to worry-that we are protected by our magnetic field-and I invited them to use the occasion to go north and enjoy the aurora that the solar wind would cause.
THE SUN'S RAREFIED corona, visible during total solar eclipses as a glowing halo around the silhouetted near side of the Moon, is a 5-million-degree plasma that is the outermost part of the solar atmosphere. With temperatures that high, the corona is the princ.i.p.al source of x-rays from the Sun, but is not otherwise visible to the human eyes. Using visible light alone, the brightness of the Sun's surface dwarfs that of the corona, easily getting lost in the glare. corona, visible during total solar eclipses as a glowing halo around the silhouetted near side of the Moon, is a 5-million-degree plasma that is the outermost part of the solar atmosphere. With temperatures that high, the corona is the princ.i.p.al source of x-rays from the Sun, but is not otherwise visible to the human eyes. Using visible light alone, the brightness of the Sun's surface dwarfs that of the corona, easily getting lost in the glare.
There's an entire layer of Earth's atmosphere where electrons have been kicked out of their host atoms by the solar wind, creating a nearby blanket of plasma we call the ionosphere. This layer reflects certain frequencies of radio waves, including those of the AM dial on your radio. Because of this property of the ionosphere, AM radio signals can reach hundreds of miles while "short wave" radio can reach thousands of miles beyond the horizon. FM signals and those of broadcast television, however, have much higher frequencies and pa.s.s right through, traveling out to s.p.a.ce at the speed of light. Any eavesdropping alien civilization will know all about our TV programs (probably a bad thing), will hear all our FM music (probably a good thing), and know nothing of the politics of AM talk-show hosts (probably a safe thing).
Most plasmas are not friendly to organic matter. The person with the most hazardous job on the Star Trek Star Trek television series is the one who must investigate the glowing blobs of plasma on the uncharted planets they visit. (My memory tells me that this person always wore a red s.h.i.+rt.) Every time this crew member meets a plasma blob, he gets vaporized. Born of the twenty-fifth century, you'd think these s.p.a.ce-faring, star-trekking people would have long ago learned to treat plasma with respect (or to not wear red). We in the twenty-first century treat plasma with respect and we haven't been anywhere. television series is the one who must investigate the glowing blobs of plasma on the uncharted planets they visit. (My memory tells me that this person always wore a red s.h.i.+rt.) Every time this crew member meets a plasma blob, he gets vaporized. Born of the twenty-fifth century, you'd think these s.p.a.ce-faring, star-trekking people would have long ago learned to treat plasma with respect (or to not wear red). We in the twenty-first century treat plasma with respect and we haven't been anywhere.
IN THE CENTER of our thermonuclear fusion reactors, where plasmas are viewed from safe distances, we attempt to bring together hydrogen nuclei at high speeds and turn them into heavier helium nuclei. In so doing, we liberate energy that could supply society's need for electricity. Problem is, we haven't yet succeeded in getting more energy out than we put in. To achieve such high collision speeds, the blob of hydrogen atoms must be raised to tens of millions of degrees. No hope for attached electrons here. At these temperatures they've all been stripped from their hydrogen atoms and roam free. How might you hold a glowing blob of hydrogen plasma at millions of degrees? In what container would you place it? Even microwave-safe Tupperware will not do. What you need is a bottle that will not melt, vaporize, or decompose. As we saw briefly in Section 2, we can use the relations.h.i.+p between plasma and magnetic fields to our advantage and design a sort of "bottle" whose walls are intense magnetic fields that the plasma cannot cross. The economic return from a successful fusion reactor will rest in part on the design of this magnetic bottle and on our understanding of how the plasma interacts with it. of our thermonuclear fusion reactors, where plasmas are viewed from safe distances, we attempt to bring together hydrogen nuclei at high speeds and turn them into heavier helium nuclei. In so doing, we liberate energy that could supply society's need for electricity. Problem is, we haven't yet succeeded in getting more energy out than we put in. To achieve such high collision speeds, the blob of hydrogen atoms must be raised to tens of millions of degrees. No hope for attached electrons here. At these temperatures they've all been stripped from their hydrogen atoms and roam free. How might you hold a glowing blob of hydrogen plasma at millions of degrees? In what container would you place it? Even microwave-safe Tupperware will not do. What you need is a bottle that will not melt, vaporize, or decompose. As we saw briefly in Section 2, we can use the relations.h.i.+p between plasma and magnetic fields to our advantage and design a sort of "bottle" whose walls are intense magnetic fields that the plasma cannot cross. The economic return from a successful fusion reactor will rest in part on the design of this magnetic bottle and on our understanding of how the plasma interacts with it.
Among the most exotic forms of matter ever concocted is the newly isolated quark-gluon plasma, created by physicists at the Brookhaven National Laboratories, a particle accelerator facility on New York's Long Island. Rather than being filled with atoms stripped of their electrons, a quark-gluon plasma comprises a mixture of the most basic const.i.tuents of matter, the fractionally charged quarks and the gluons that normally hold them together to make protons and neutrons themselves. This unusual form of plasma resembles greatly the state of the entire universe, a fraction of a second after the big bang. This was about the time the observable universe could still fit within the 87-foot sphere of the Rose Center for Earth and s.p.a.ce. Indeed, in one form or another, every cubic inch of the universe was in a plasma state until nearly 400,000 years had elapsed.
Until then, the universe had cooled from trillions of degrees down to a few thousand. The whole time, all light was scattered left and right by the free electrons of our plasma-filled universe-a state that greatly resembles what happens to light as it pa.s.ses through frosted gla.s.s or the Sun's interior. Light can travel through neither without scattering, rendering them both translucent instead of transparent. Below a few thousand degrees, the universe cooled enough for every electron in the cosmos to combine with one atomic nuclei, creating complete atoms of hydrogen and helium.
The pervasive plasma state no longer existed as soon as every electron found a home. And that's the way it would stay for hundreds of millions of years, at least until quasars were born, with their central black holes that dine on swirling gases. Just before the gas falls in, it releases ionizing ultraviolet light that travels across the universe, kicking electrons back out of their atoms with abandon. Until the quasars were born, the universe had enjoyed the only interval of time (before or since) where plasma was nowhere to be found. We call this era the Dark Ages and look upon it as a time when gravity was silently and invisibly a.s.sembling matter into plasma b.a.l.l.s that became the first generation of stars.
NINETEEN.
FIRE AND ICE.
When Cole Porter composed "Too Darn Hot" for his 1948 Broadway musical Kiss Me Kate Kiss Me Kate, the temperature he was bemoaning was surely no higher than the mid-nineties. No harm in taking Porter's lyrics as an authoritative source on the upper temperature limit for comfortable lovemaking. Combine that with what a cold shower does to most people's erotic urges, and you now have a pretty good estimate of how narrow the comfort zone is for the unclothed human body: a range of about 30 degrees Fahrenheit, with room temperature just about in the middle.
The universe is a whole other story. How does a temperature of 100,000,000,000,000,000,000,000,000,000,000 degrees grab you? That's a hundred thousand billion billion billion degrees. It also happens to be the temperature of the universe a teeny fraction of a second after the big bang-a time when all the energy and matter and s.p.a.ce that would turn into planets, petunias, and particle physicists was an expanding fiery ball of quark-gluon plasma. Nothing you'd call a thing could exist until there was a multibillion-fold cooling of the cosmos.
As the laws of thermodynamics decree, within about one second after the big bang, the expanding fireball had cooled to 10 billion degrees and ballooned from something smaller than an atom to a cosmic colossus about a thousand times the size of our solar system. By the time three minutes had pa.s.sed, the universe was a balmy billion degrees and was already hard at work making the simplest atomic nuclei. Expansion is the handmaiden to cooling, and the two have continued, unabated, ever since.
Today the average temperature of the universe is 2.73 degrees Kelvin. All the temperatures mentioned so far, aside from the ones that involve the human libido, are stated in degrees Kelvin. The Kelvin degree, known simply as the kelvin, was conceived to be the same temperature interval as the Celsius degree, but the Kelvin scale has no negative numbers. Zero is zero, period. In fact, to quash all doubts, zero on the Kelvin scale is dubbed absolute zero.
The Scottish engineer and physicist William Thomson, later and better known as Lord Kelvin, first articulated the idea of a coldest possible temperature in 1848. Laboratory experiments haven't gotten there yet. As a matter of principle, they never will, although they've come awfully close. The unarguably cold temperature of 0.0000000005 K (or 500 pic.o.kelvins, as metric mavens would say) was artfully achieved in 2003 in the lab of Wolfgang Ketterle, a physicist at MIT.
Outside the laboratory, cosmic phenomena span a staggering range of temperatures. Among the hottest places in the universe today is the core of a blue supergiant star during the hours of its collapse. Just before it explodes as a supernova, creating drastic neighborhood-warming effects, its temperature hits 100 billion K. Compare that with the Sun's core: a mere 15 million K.
Surfaces are much cooler. The skin of a blue supergiant checks in at about 25,000 K-hot enough, of course, to glow blue. Our Sun registers 6,000 K-hot enough to glow white, and hot enough to melt and then vaporize anything in the periodic table of elements. The surface of Venus is 740 K, hot enough to fry the electronics normally used to drive s.p.a.ce probes.
Considerably further down the scale is the freezing point of water, 273.15 K, which looks downright warm compared with the 60 K surface of Neptune, nearly 3 billion miles from the Sun. Colder still is Triton, one of Neptune's moons. Its icy nitrogen surface sinks to 40 K, making it the coldest place in the solar system this side of Pluto.
Where do Earth-beings fit in? The average body temperature of humans (traditionally 98.6 degrees F) registers slightly above 310 on the Kelvin scale. Officially recorded surface temperatures on Earth range from a summer high of 331 K (136 F, at Al 'Aziziyah, Libya, in 1922) to a winter low of 184 K (129 F, at Base Vostok, Antarctica, in 1983). But people can't survive una.s.sisted at those extremes. We suffer hyperthermia in the Sahara if we don't have shelter from the heat, and hypothermia in the Arctic if we don't have boatloads of clothing and caravans of food. Meanwhile, Earth-dwelling extremophile microorganisms, both thermophilic (heat-loving) and psychrophilic (cold-loving), are variously adapted to temperatures that would fry us or freeze us. Viable yeast, wearing no clothes at all, has been discovered in 3-million-year-old Siberian permafrost. A species of bacterium locked in Alaskan permafrost for 32,000 years woke up and started swimming as soon as its medium melted. And at this very moment, a.s.sorted species of archaea and bacteria are living out their lives in boiling mud, bubbling hot springs, and undersea volcanoes.
Even complex organisms can survive in similarly astonis.h.i.+ng circ.u.mstances. When provoked, the itsy-bitsy invertebrates known as tardigrades can suspend their metabolism. In that state, they can survive temperatures of 424 K (303 degrees F) for several minutes and 73 K (328 degrees F) for days on end, making them hardy enough to endure being stranded on Neptune. So the next time you need s.p.a.ce travelers with the "right stuff," you might want to choose yeast and tardigrades, and leave your astronauts, cosmonauts, and taikonauts* at home. at home.
IT'S COMMON TO confuse temperature with heat. Heat is the total energy of all the motions of all the molecules in your substance of choice. It so happens that, within the mixture, the range of energies is large: some molecules move quickly, others move slowly. Temperature simply measures their average energy. For example, a cup of freshly brewed coffee may have a higher temperature than a heated swimming pool, but all the water in the pool holds vastly more heat than does the lone cup of coffee. If you rudely pour your 200-degree coffee into the 100-degree pool, the pool won't suddenly become 150 degrees. And whereas two people in a bed are a source of twice as much heat as one person in a bed, the average temperatures of their two bodies-98.6 and 98.6-do not normally add up to an undercover oven whose temperature is 197.2 degrees. confuse temperature with heat. Heat is the total energy of all the motions of all the molecules in your substance of choice. It so happens that, within the mixture, the range of energies is large: some molecules move quickly, others move slowly. Temperature simply measures their average energy. For example, a cup of freshly brewed coffee may have a higher temperature than a heated swimming pool, but all the water in the pool holds vastly more heat than does the lone cup of coffee. If you rudely pour your 200-degree coffee into the 100-degree pool, the pool won't suddenly become 150 degrees. And whereas two people in a bed are a source of twice as much heat as one person in a bed, the average temperatures of their two bodies-98.6 and 98.6-do not normally add up to an undercover oven whose temperature is 197.2 degrees.
Scientists in the seventeenth and eighteenth centuries considered heat to be closely linked with combustion. And combustion, as they understood it, happened when phlogiston, a hypothetical earthlike substance characterized mainly by its combustibility, was removed from an object. Burn a log in the fireplace, air carries off the phlogiston, and the dephlogisticated log reveals itself as a pile of ashes.
By the late eighteenth century the French chemist Antoine-Laurent Lavoisier had replaced phlogiston theory with caloric theory. Lavoisier cla.s.sified heat, which he called caloric, as one of the chemical elements, and contended that it was an invisible, tasteless, odorless, weightless fluid that pa.s.sed between objects through combustion or rubbing. The concept of heat was not fully understood until the nineteenth century, the peak of the industrial revolution, when the broader concept of energy took shape within the new branch of physics called thermodynamics.
ALTHOUGH HEAT as a scientific idea posed plenty of challenges to brilliant minds, both scientists and nonscientists have intuitively grasped the concept of temperature for millennia. Hot things have a high temperature. Cold things have a low temperature. Thermometers confirm the connection. as a scientific idea posed plenty of challenges to brilliant minds, both scientists and nonscientists have intuitively grasped the concept of temperature for millennia. Hot things have a high temperature. Cold things have a low temperature. Thermometers confirm the connection.
Although Galileo is often credited with the invention of the thermometer, the earliest such device may have been built by the first-century A.D A.D. inventor Heron of Alexandria. Heron's book Pneumatica Pneumatica includes a description of a "thermoscope," a device that showed the change in the volume of a gas as it was heated or cooled. Like many other ancient texts, includes a description of a "thermoscope," a device that showed the change in the volume of a gas as it was heated or cooled. Like many other ancient texts, Pneumatica Pneumatica was translated into Latin during the Renaissance. Galileo read it in 1594 and, as he later did when he learned of the newly invented telescope, he immediately constructed a better thermoscope. Several of his contemporaries did the same. was translated into Latin during the Renaissance. Galileo read it in 1594 and, as he later did when he learned of the newly invented telescope, he immediately constructed a better thermoscope. Several of his contemporaries did the same.
For a thermometer, scale is crucial. There's a curious tradition, beginning early in the eighteenth century, of calibrating the temperature units in such a way that common phenomena get a.s.signed fraction-friendly numbers with many divisors. Isaac Newton proposed a scale from zero (melting snow) to 12 (the human body); 12 is, of course, evenly divisible by 2, 3, 4, and 6. The Danish astronomer Ole Rmer offered a scale from zero to 60 (60 being divisible by 2, 3, 4, 5, 6, 10, 12, 15, 20, and 30). On Rmer's scale, zero was the lowest temperature he could achieve with a mixture of ice, salt, and water; 60 was the boiling point of water.
In 1724 a German instrument maker named Daniel Gabriel Fahrenheit (who developed the mercury thermometer in 1714) came up with a more precise scale, splitting each degree of Rmer's into four equal parts. On the new scale, water boiled at 240 degrees and froze at 30, and human body temperature was about 90. After further adjustments, the span from zero to body temperature became 96 degrees, another winner in the divisibility department (its divisors are 2, 3, 4, 6, 8, 12, 16, 24, 32, and 48). The freezing point of water became 32 degrees. Still further tuning and standardization saddled fans of the Fahrenheit scale with a body temperature that isn't a round number, and a boiling point of 212 degrees.
Following a different path, in 1742 the Swedish astronomer Anders Celsius proposed a decimal-friendly centigrade scale for temperature. He set the freezing point at 100 and the boiling point at zero. This was not the first or last time an astronomer labeled a scale backward. Somebody, quite possibly the chap who manufactured Celsius's thermometers, did the world a favor and reversed the numbering, giving us the now-familiar Celsius scale. The number zero seems to have a crippling effect on some people's comprehension. One night a couple of decades ago, while I was on winter break from graduate school and was staying at my parents' house north of New York City, I turned on the radio to listen to cla.s.sical music. A frigid Canadian air ma.s.s was advancing on the Northeast, and the announcer, between movements of George Frideric Handel's Water Music, continually tracked the descending outdoor temperature: "Five degrees Fahrenheit." "Four degrees." "Three degrees." Finally, sounding distressed, he announced, "If this keeps up, pretty soon there'll be no temperature left!"
In part to avoid such embarra.s.sing examples of innumeracy, the international community of scientists uses the Kelvin temperature scale, which puts zero in the right place: at the absolute bottom. Any other location for zero is arbitrary and does not lend itself to play-by-play arithmetic commentary.
Several of Kelvin's predecessors, by measuring the shrinking volume of a gas as it cooled, had established273.15 degrees Celsius (459.67 degrees F) as the temperature at which the molecules of any substance have the least possible energy. Other experiments showed that273.15 C is the temperature at which a gas, when kept at constant pressure, would drop to zero volume. Since there is no such thing as a gas with zero volume,273.15 C became the unattainable lower limit of the Kelvin scale. And what better term to use for it than "absolute zero"?
THE UNIVERSE AS a whole acts somewhat like a gas. If you force a gas to expand, it cools. Back when the universe was a mere half-million years old, the cosmic temperature was about 3,000 K. Today it is less than 3 K. Inexorably expanding toward thermal oblivion, the present-day universe is a thousand times larger, and a thousand times cooler, than the infant universe. a whole acts somewhat like a gas. If you force a gas to expand, it cools. Back when the universe was a mere half-million years old, the cosmic temperature was about 3,000 K. Today it is less than 3 K. Inexorably expanding toward thermal oblivion, the present-day universe is a thousand times larger, and a thousand times cooler, than the infant universe.
On Earth, you normally measure temperatures by cramming a thermometer into a creature's orifice or letting the thermometer touch an object in some other, less intrusive way. This form of direct contact enables the moving molecules within the thermometer to reach the same average energy as the molecules in the object. When a thermometer sits idle in the air instead of performing its labors inside a rib roast, it's the average speed of the colliding air molecules that tell the thermometer what temperature to register.
Speaking of air, at a given time and place on Earth the air temperature in full sunlight is basically the same as the air temperature under a nearby tree. What the shade does is s.h.i.+eld you from the Sun's radiant energy, nearly all of which pa.s.ses unabsorbed through the atmosphere and lands on your skin, making you feel hotter than the air would by itself. But in empty s.p.a.ce, where there is no air, there are no moving molecules to trigger a thermometer reading. So the question "What is the temperature of s.p.a.ce?" has no obvious meaning. With nothing touching it, the thermometer can only register the radiant energy from all the light, from all sources, that lands upon it.
On the daytime side of our airless Moon, a thermometer would register 400 K (260 degrees F). Move a few feet into the shadow of a boulder, or journey to the Moon's night side, and the thermometer would instantly drop to 40 K (390 degrees F). To survive a lunar day without wearing a temperature-controlled s.p.a.ce suit, you would have to do pirouettes, alternately baking and then cooling all sides of your body, just to maintain a comfortable temperature.
WHEN THE GOING gets really cold and you want to absorb maximum radiant energy, wear something dark rather than reflective. The same holds for a thermometer. Rather than debate how to dress it in s.p.a.ce, a.s.sume the thermometer can be made perfectly absorbent. If you now place it in the middle of nowhere, such as halfway between the Milky Way and the Andromeda galaxy, far from all obvious sources of radiation, the thermometer will settle at 2.73 K, the current background temperature of the universe. gets really cold and you want to absorb maximum radiant energy, wear something dark rather than reflective. The same holds for a thermometer. Rather than debate how to dress it in s.p.a.ce, a.s.sume the thermometer can be made perfectly absorbent. If you now place it in the middle of nowhere, such as halfway between the Milky Way and the Andromeda galaxy, far from all obvious sources of radiation, the thermometer will settle at 2.73 K, the current background temperature of the universe.
A recent consensus among cosmologists holds that the universe will expand forever and ever. By the time the cosmos doubles in size, its temperature will drop by half. By the time it doubles again, its temperature will halve once more. With the pa.s.sage of trillions of years, all the remaining gas will have been used to make stars, and all the stars will have exhausted their thermonuclear fuels. Meanwhile, the temperature of the expanding universe will continue to descend, approaching ever closer to absolute zero.
SECTION 4.
THE MEANING OF LIFE.
THE CHALLENGES AND TRIUMPHS OF KNOWING HOW WE GOT HERE.
TWENTY.
DUST TO DUST.
Acasual look at the Milky Way with the unaided eye reveals a cloudy band of light and dark splotches extending from horizon to horizon. With the help of simple binoculars or a backyard telescope, the dark and boring areas of the Milky Way resolve into, well, dark and boring areas-but the bright areas resolve into countless stars and nebulae.
In a small book ent.i.tled Sidereus Nuncius Sidereus Nuncius (The Starry Messenger), published in Venice in 1610, Galileo gives an account of the heavens as seen through a telescope, including the first-ever description of the Milky Way's patches of light. Referring to his yet-to-be-named instrument as a "spygla.s.s," he is so excited he can barely contain himself: (The Starry Messenger), published in Venice in 1610, Galileo gives an account of the heavens as seen through a telescope, including the first-ever description of the Milky Way's patches of light. Referring to his yet-to-be-named instrument as a "spygla.s.s," he is so excited he can barely contain himself: The Milky Way itself, which, with the aid of the spygla.s.s, may be observed so well that all the disputes that for so many generations have vexed philosophers are destroyed by visible certainty, and we are liberated from wordy arguments. For the Galaxy is nothing else than a congeries of innumerable stars distributed in cl.u.s.ters. To whatever region of it you direct your spygla.s.s, an immense number of stars immediately offer themselves to view, of which very many appear rather large and very conspicuous but the mult.i.tude of small ones is truly unfathomable. (Van Helden 1989, p. 62) (Van Helden 1989, p. 62) Surely "immense number of stars" is where the action is. Why would anybody be interested in the dark areas where stars are absent? They are probably cosmic holes to the infinite and empty beyond.
Three centuries would pa.s.s before anybody figured out that the dark patches are thick, dense clouds of gas and dust, which obscure the more distant star fields and hold stellar nurseries deep within. Following earlier suppositions of the American astronomer George Cary Comstock, who wondered why faraway stars were much dimmer than their distance alone would indicate, it was not until 1909 when the Dutch astronomer Jacobus Cornelius Kapteyn (18511922) would name the culprit. In two research papers, both t.i.tled "On the Absorption of Light in s.p.a.ce," Kapteyn presented evidence that clouds, his newfound "interstellar medium," not only scatter the overall light of stars but do so unevenly across the rainbow of colors in a star's spectrum, attenuating the blue light more severely than the red. This selective absorption makes the Milky Way's faraway stars look, on average, redder than the near ones.
Ordinary hydrogen and helium, the princ.i.p.al const.i.tuents of cosmic gas clouds, don't redden light. But larger molecules do-especially those that contain the elements carbon and silicon. And when the molecules get too big to be called molecules, we call them dust.
Death By Black Hole Part 7
You're reading novel Death By Black Hole Part 7 online at LightNovelFree.com. You can use the follow function to bookmark your favorite novel ( Only for registered users ). If you find any errors ( broken links, can't load photos, etc.. ), Please let us know so we can fix it as soon as possible. And when you start a conversation or debate about a certain topic with other people, please do not offend them just because you don't like their opinions.
Death By Black Hole Part 7 summary
You're reading Death By Black Hole Part 7. This novel has been translated by Updating. Author: Neil deGrasse Tyson already has 516 views.
It's great if you read and follow any novel on our website. We promise you that we'll bring you the latest, hottest novel everyday and FREE.
LightNovelFree.com is a most smartest website for reading novel online, it can automatic resize images to fit your pc screen, even on your mobile. Experience now by using your smartphone and access to LightNovelFree.com
- Related chapter:
- Death By Black Hole Part 6
- Death By Black Hole Part 8
RECENTLY UPDATED NOVEL

Quick Transmigration Cannon Fodder's Record of Counterattacks
Quick Transmigration Cannon Fodder's Record of Counterattacks Chapter 2911: The Higher You Climb, the Harder You Fall View : 2,894,369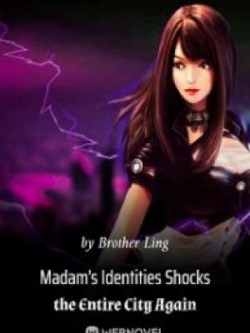
Madam's Identities Shocks The Entire City Again
Madam's Identities Shocks The Entire City Again Chapter 3429: Xie Yun: I Don't Object View : 2,649,709
The Death Mage Who Doesn't Want a Fourth Time
The Death Mage Who Doesn't Want a Fourth Time Chapter 374: Parting with a season of encounters and partings, and a blank check View : 320,940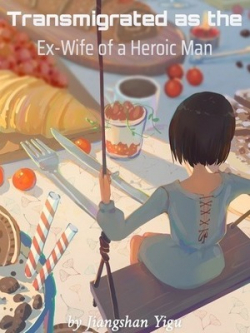
Transmigrated as the Ex-Wife of a Heroic Man
Transmigrated as the Ex-Wife of a Heroic Man Chapter 1283: Playing the Bad Guy View : 557,066