On Food And Cooking Part 100
You’re reading novel On Food And Cooking Part 100 online at LightNovelFree.com. Please use the follow button to get notification about the latest chapter next time when you visit LightNovelFree.com. Use F11 button to read novel in full-screen(PC only). Drop by anytime you want to read free – fast – latest novel. It’s great if you could leave a comment, share your opinion about the new chapters, new novel with others on the internet. We’ll do our best to bring you the finest, latest novel everyday. Enjoy!
Proteins in Water In living systems and in most foods, protein molecules are surrounded by water. Because all proteins are capable to some extent of hydrogen bonding, they absorb and hold at least some water, although the amounts vary greatly according to the kinds of side groups present and the overall structure of the molecule. Water molecules can be held "inside" the protein, along the backbone, and "outside," on polar side groups.
Whether or not a protein is soluble soluble in water depends on the strength of the bonds between molecules, and on whether water can separate the molecules from each other by hydrogen bonding. The wheat proteins that form gluten when flour is mixed with water are a kind of protein that absorbs considerable amounts of water but doesn't dissolve, because many fat-like groups along their molecules bond with each other, hold the proteins together, and exclude water. Similarly, the proteins that make up the contracting muscle fibers in meat are held together by ionic and other bonds. On the other hand, many of the proteins in milk and eggs are quite soluble. in water depends on the strength of the bonds between molecules, and on whether water can separate the molecules from each other by hydrogen bonding. The wheat proteins that form gluten when flour is mixed with water are a kind of protein that absorbs considerable amounts of water but doesn't dissolve, because many fat-like groups along their molecules bond with each other, hold the proteins together, and exclude water. Similarly, the proteins that make up the contracting muscle fibers in meat are held together by ionic and other bonds. On the other hand, many of the proteins in milk and eggs are quite soluble.
Protein Denaturation A very important characteristic of proteins is their susceptibility to denaturation, denaturation, or the undoing of their natural structure by chemical or physical means. This change involves breaking the bonds that maintain the molecule's folded shape. (The strong backbone bonds are broken only in extreme conditions or with the help of enzymes.) Denaturation is not a change in composition, only a change in structure. But structure determines behavior, and denatured proteins behave very differently from their originals. or the undoing of their natural structure by chemical or physical means. This change involves breaking the bonds that maintain the molecule's folded shape. (The strong backbone bonds are broken only in extreme conditions or with the help of enzymes.) Denaturation is not a change in composition, only a change in structure. But structure determines behavior, and denatured proteins behave very differently from their originals.
Proteins can be denatured in many ways: by exposing them to heat - usually to somewhere between 140180F/6080C - or to high acidity, or to air bubbles, or to a combination of these. In each case, the unusual chemical or physical conditions - increased molecular agitation, or lots of reactive protons, or the drastic difference between the air bubble and the liquid wall that surrounds it - breaks many of the bonds between amino acid side groups that hold the protein molecule in its specific folded shape. The long proteins therefore unfold, exposing many more of their reactive side groups to the watery environment.
Protein Coagulation There are several general consequences of denaturation that follow for most food proteins. Because the molecules have been extended in length, they're more likely to b.u.mp into each other. And because their side groups are now exposed and available for forming bonds, denatured proteins begin to bond with each other, or There are several general consequences of denaturation that follow for most food proteins. Because the molecules have been extended in length, they're more likely to b.u.mp into each other. And because their side groups are now exposed and available for forming bonds, denatured proteins begin to bond with each other, or coagulate. coagulate. This happens throughout the food, and results in the development of a continuous network of proteins, with water held in the pockets between protein strands. The food therefore develops a kind of thickness or density that can be delicate and delightful, as in a barely set custard or perfectly cooked piece of fish. However, if cooking or other denaturing conditions continue, given the extreme physical or chemical environment that caused the proteins to denature in the first place, only the stronger bonds can form and survive, which means that the proteins bond together more and more tightly, densely, and irreversibly. And as they do so, they squeeze the pockets of water out from between them. The custard gets dense and a watery fluid separates from the solid portion; the fish gets tough and dry. This happens throughout the food, and results in the development of a continuous network of proteins, with water held in the pockets between protein strands. The food therefore develops a kind of thickness or density that can be delicate and delightful, as in a barely set custard or perfectly cooked piece of fish. However, if cooking or other denaturing conditions continue, given the extreme physical or chemical environment that caused the proteins to denature in the first place, only the stronger bonds can form and survive, which means that the proteins bond together more and more tightly, densely, and irreversibly. And as they do so, they squeeze the pockets of water out from between them. The custard gets dense and a watery fluid separates from the solid portion; the fish gets tough and dry.
The details of protein denaturation and coagulation in any given food are intricate and fascinating. For example, acidity and salts can cause egg proteins to cl.u.s.ter together even before they begin to unfold, and thus affect the consistency of scrambled eggs and custards. Such details are noted in the descriptions of particular foods.
Enzymes There's a particular group of proteins that are important to the cook not so much for their direct contribution to food texture and consistency, but for the way they change the other components of the food they're in. These proteins are the enzymes. enzymes. Enzymes are biological catalysts: that is, they increase the rate of specific chemical reactions that otherwise would occur only very slowly, if at all. Enzymes thus cause chemical change. Some enzymes build molecules up, or modify them; some break molecules down. Human digestive enzymes, for example, break proteins into individual amino acids, and starch into individual glucose units. A singe enzyme molecule can catalyze as many as a million reactions per second. Enzymes are biological catalysts: that is, they increase the rate of specific chemical reactions that otherwise would occur only very slowly, if at all. Enzymes thus cause chemical change. Some enzymes build molecules up, or modify them; some break molecules down. Human digestive enzymes, for example, break proteins into individual amino acids, and starch into individual glucose units. A singe enzyme molecule can catalyze as many as a million reactions per second.
Enzymes matter to the cook because foods contain enzymes that once did important work for the plant or animal when it was alive, but that can now harm the food by changing its color, texture, taste, or nutritiousness. Enzymes help turn green chlorophyll in vegetables dull olive, cause cut fruits to turn brown and oxidize their vitamin C, and turn fish flesh mushy. And bacterial spoilage is largely a matter of bacterial enzymes breaking the food down for the bacteria's own use. With a few exceptions - the tenderizing of meat by its own internal enzymes, the firming of some vegetables before further cooking, and fermentations in general - the cook wants to prevent enzymatic activity in food. Storing foods at low temperatures delays spoilage in part because it slows the growth of spoilage microbes, but also because it slows the activity of the food's own enzymes.
Cooking Accelerates Enzyme Action Before Stopping It Because the activity of an enzyme depends on its structure, any change in that structure will destroy its effectiveness. So cooking foods sufficiently will denature and inactivate any enzymes they may contain. One vivid example of this principle is the behavior of raw and cooked pineapple in gelatin. Pineapples and certain other fruits contain an enzyme that breaks proteins down into small fragments. If raw pineapple is combined with gelatin to make a jelly, the enzyme digests the gelatin molecules and liquifies the jelly. But canned pineapple has been heated enough to denature the enzyme, and makes a firm gelatin jelly. Because the activity of an enzyme depends on its structure, any change in that structure will destroy its effectiveness. So cooking foods sufficiently will denature and inactivate any enzymes they may contain. One vivid example of this principle is the behavior of raw and cooked pineapple in gelatin. Pineapples and certain other fruits contain an enzyme that breaks proteins down into small fragments. If raw pineapple is combined with gelatin to make a jelly, the enzyme digests the gelatin molecules and liquifies the jelly. But canned pineapple has been heated enough to denature the enzyme, and makes a firm gelatin jelly.
There's a complication, though. The reactivity of most chemicals increases with increasing temperature. The rule of thumb is that reactivity doubles with each rise of 20F/10C. The same tendency goes for enzymes, up to a range in which they begin to denature, become less effective, and finally become completely inactive. This means that cooking gives enzymes a chance to do their damage more and more quickly as the temperature rises, and only stops them once they reach their denaturation temperature. In general, the best rule is to heat foods as rapidly as possible, thereby minimizing the period during which the enzymes are at their optimum temperatures, and to get them all the way to the boiling point. Conversely, desirable enzyme action - meat tenderizing, for example - can be maximized by slow, gradual heating to denaturing temperatures.
Appendix A Chemistry Primer Atoms, Molecules, Energy
Atoms, Molecules, and Chemical Bonds Atoms and MoleculesElectrical Imbalance, Reactions, and OxidationElectrical Imbalance and Chemical Bonds Energy Energy Causes ChangeThe Nature of Heat: Molecular MovementBond Energy The Phases of Matter SolidsLiquidsGasesMany Food Molecules Can't Change PhaseMixtures of Phases: Solutions, Suspensions, Emulsions, Gels, Foams Cooking is applied chemistry, and the basic concepts of chemistry - molecules, energy, heat, reactions - are keys to a clearer understanding of what our foods are and how we transform them. A casual acquaintance with these concepts is enough to follow most of the explanations in this book. For readers who'd like to get to know them better, here's a brief review.
Atoms, Molecules, and Chemical Bonds It was the ancient Greeks who gave us the idea of atoms, atoms, fundamental and invisibly small particles of matter, and also the word fundamental and invisibly small particles of matter, and also the word atom, atom, which means "uncuttable," "indivisible." Greek philosophers proposed that there are just four basic kinds of particles in the world - atoms of earth, air, water, and fire - and that all material things, our bodies and our foods and everything else, are built from these primary particles. The modern scientific view of matter's invisible innards is more complicated, but also more precise and illuminating. which means "uncuttable," "indivisible." Greek philosophers proposed that there are just four basic kinds of particles in the world - atoms of earth, air, water, and fire - and that all material things, our bodies and our foods and everything else, are built from these primary particles. The modern scientific view of matter's invisible innards is more complicated, but also more precise and illuminating.
Atoms and Molecules All matter on earth is a mixture of around 100 pure substances, which we call the elements elements: hydrogen, oxygen, nitrogen, carbon, and so on. An atom is the smallest particle into which an element can be subdivided without losing its characteristic properties. Atoms are very small indeed: several million would fit into the period at the end of this sentence. All atoms are made up of smaller "subatomic" particles, electrons, protons, electrons, protons, and and neutrons. neutrons. The different properties of the elements arise from the varying combinations of subatomic particles that make up their atoms, and in particular their quotas of protons and electrons. Hydrogen atoms contain one proton and one electron; oxygen carries 8 of each, and iron 26. The different properties of the elements arise from the varying combinations of subatomic particles that make up their atoms, and in particular their quotas of protons and electrons. Hydrogen atoms contain one proton and one electron; oxygen carries 8 of each, and iron 26.
When two or more atoms bond together, which they do by sharing electrons with each other, they form a molecule molecule (from the Latin for "ma.s.s," "bulk"). The molecule is to a chemical compound what the atom is to an element: the smallest unit that retains the properties of the original material. Most matter on earth, including food, is a mixture of different chemical compounds. (from the Latin for "ma.s.s," "bulk"). The molecule is to a chemical compound what the atom is to an element: the smallest unit that retains the properties of the original material. Most matter on earth, including food, is a mixture of different chemical compounds.
Protons and Electrons Carry Positive and Negative Electrical Charges There's one primary driving force behind all the chemical activity that makes life and cooking possible, and that's the electrical attraction between protons and electrons. Protons carry a positive electrical charge, and electrons an exactly balancing negative charge. (The neutral neutron carries no charge.) Opposite electrical charges attract each other; similar electrical charges repel each other. In each atom, protons in the central nucleus attract a cloud of electrons that orbit constantly at various distances from the nucleus. Stable forms of the elements are electrically neutral, which means that their atoms contain equal numbers of protons and electrons. There's one primary driving force behind all the chemical activity that makes life and cooking possible, and that's the electrical attraction between protons and electrons. Protons carry a positive electrical charge, and electrons an exactly balancing negative charge. (The neutral neutron carries no charge.) Opposite electrical charges attract each other; similar electrical charges repel each other. In each atom, protons in the central nucleus attract a cloud of electrons that orbit constantly at various distances from the nucleus. Stable forms of the elements are electrically neutral, which means that their atoms contain equal numbers of protons and electrons.
(If like charges repel each other and opposite charges attract, then why is it that the protons in the nucleus don't push each other away and the orbiting electrons fall straight into the nucleus? It turns out that there are forces besides electricity at work in the atom. The protons and neutrons are bound together by very strong nuclear forces, while it's the nature of electrons to be in continual motion. So protons and electrons are always attracted to each other and move in response to the other's presence, but they never consummate their attraction.) Electrical Imbalance, Reactions, and Oxidation The electrons in atoms are arranged around the nucleus in orbits that determine how strongly any particular electron is held there. Some electrons are held close and tightly to the nucleus, while others range far away and are held more weakly. The behavior of the outermost electrons largely determines the chemical behavior of the elements. For example, the elements cla.s.si-fied as metals - copper, aluminum, iron - hold their outermost electrons very weakly, and easily give them away to the atoms of other elements - oxygen, chlorine - that are hungrier for electrons, and that tend to grab up any that are loosely held. This imbalance in electrical pulls among different elements is the basis of most chemical reactions. chemical reactions. Reactions are encounters among atoms and molecules that result in the loss, gain, or sharing of electrons, and thus changes in the properties of the atoms and molecules involved. Reactions are encounters among atoms and molecules that result in the loss, gain, or sharing of electrons, and thus changes in the properties of the atoms and molecules involved.
An atom of carbon. Carbon has six protons and six neutrons in its nucleus, and six electrons...o...b..ting around the nucleus.
Of all the electron-grabbing elements, the most important is oxygen, so much so that chemists use the term oxidation oxidation to name the general chemical activity of grabbing electrons from other atoms, even if a chlorine atom is doing the grabbing. Oxidation is very important in the kitchen, because oxygen is always present in the air, and readily robs electrons from the carbon-hydrogen chains of fats, oils, and aroma molecules. This initial oxidation triggers a cascade of further oxidations and other reactions that end up breaking the original large fat molecules into small, strong-smelling fragments. to name the general chemical activity of grabbing electrons from other atoms, even if a chlorine atom is doing the grabbing. Oxidation is very important in the kitchen, because oxygen is always present in the air, and readily robs electrons from the carbon-hydrogen chains of fats, oils, and aroma molecules. This initial oxidation triggers a cascade of further oxidations and other reactions that end up breaking the original large fat molecules into small, strong-smelling fragments. Antioxidant Antioxidant substances - for example, phenolic compounds found in many foods made from plants - prevent this breakdown by giving oxygen the electrons it wants without starting a reaction cascade, thus sparing the fat molecules from oxidation. substances - for example, phenolic compounds found in many foods made from plants - prevent this breakdown by giving oxygen the electrons it wants without starting a reaction cascade, thus sparing the fat molecules from oxidation.
Electrical Imbalance and Chemical Bonds Electron hunger is also the basis for the chemical bond, chemical bond, an interaction between atoms or molecules that holds them together, either loosely or tightly, momentarily or indefinitely. There are several different kinds of chemical bonds that are important in the kitchen, as they are throughout nature. an interaction between atoms or molecules that holds them together, either loosely or tightly, momentarily or indefinitely. There are several different kinds of chemical bonds that are important in the kitchen, as they are throughout nature.
Ionic Bonds; Salt One kind of chemical bond is the One kind of chemical bond is the ionic bond, ionic bond, in which one atom completely captures the electron(s) of another, so great is the difference between their electron hungers. Chemical compounds held together by ionic bonds don't simply dissolve in water; they come apart into separate in which one atom completely captures the electron(s) of another, so great is the difference between their electron hungers. Chemical compounds held together by ionic bonds don't simply dissolve in water; they come apart into separate ions, ions, or atoms that are electrically charged because they either carry extra electrons or gave up some of their electrons. (The term was coined by the pioneer of electricity, Michael Faraday, from the Greek word for "going," to name those electrically charged particles that move when an electrical field is set up in a water solution.) Salt, our most common seasoning, is a compound of sodium and chlorine held together with ionic bonds. In a solid crystal of pure salt, positively charged sodium ions alternate with negatively charged chloride ions, the sodiums having lost their electrons to the chlorines. Because several positive sodium ions are always in a state of attraction to several negative chloride ions, we can't really speak of individual molecules of salt, with one particular sodium atom bonded to a particular chlorine atom. In water, salt dissolves into separate positive sodium ions and negative chloride ions. or atoms that are electrically charged because they either carry extra electrons or gave up some of their electrons. (The term was coined by the pioneer of electricity, Michael Faraday, from the Greek word for "going," to name those electrically charged particles that move when an electrical field is set up in a water solution.) Salt, our most common seasoning, is a compound of sodium and chlorine held together with ionic bonds. In a solid crystal of pure salt, positively charged sodium ions alternate with negatively charged chloride ions, the sodiums having lost their electrons to the chlorines. Because several positive sodium ions are always in a state of attraction to several negative chloride ions, we can't really speak of individual molecules of salt, with one particular sodium atom bonded to a particular chlorine atom. In water, salt dissolves into separate positive sodium ions and negative chloride ions.
Ionic and covalent bonds. Left: Left: An ionic bond results when an atom completely captures one or more electrons of another atom, and the two atoms experience an attractive force (dotted line) due to their opposite electrical charges. An ionic bond results when an atom completely captures one or more electrons of another atom, and the two atoms experience an attractive force (dotted line) due to their opposite electrical charges. Right: Right: In the covalent bond, atoms share electrons, and thereby form stable combinations called molecules. In the covalent bond, atoms share electrons, and thereby form stable combinations called molecules.
Strong Bonds That Make Molecules A second kind of chemical bond, called A second kind of chemical bond, called covalent covalent (from the Latin, "of equal power"), produces stable (from the Latin, "of equal power"), produces stable molecules. molecules. When two atoms have roughly similar affinities for electrons, they will When two atoms have roughly similar affinities for electrons, they will share share them rather than gain or lose them entirely. In order for sharing to occur, the electron clouds of two atoms must overlap, and this condition results in a fixed arrangement in s.p.a.ce between two particular atoms, which thus form a stable combined structure. The bonding geometry determines the overall shape of the molecule, and molecular shape in turn defines the ways in which one molecule can react with others. them rather than gain or lose them entirely. In order for sharing to occur, the electron clouds of two atoms must overlap, and this condition results in a fixed arrangement in s.p.a.ce between two particular atoms, which thus form a stable combined structure. The bonding geometry determines the overall shape of the molecule, and molecular shape in turn defines the ways in which one molecule can react with others.
The elements most important to life on earth - hydrogen, oxygen, carbon, nitrogen, phosphorus, sulfur - all tend to form covalent bonds, and these make possible the complex, stable a.s.semblages that const.i.tute our bodies and our foods. The most familiar pure chemical compounds in the kitchen are water, a covalent combination of two hydrogen atoms and an oxygen; and sucrose, or table sugar, a combination of carbon, oxygen, and hydrogen atoms. Covalent bonds are generally strong and stable at room temperature: that is, they're not broken in significant numbers unless subjected to heat or to reactive chemicals, including enzymes. Unlike salt, which dissolves into electrically charged ions, covalently bonded molecules that can dissolve in water generally do so as intact, electrically neutral molecules.
Weak Bonds Between Polar Molecules: Water A third kind of chemical bond, about a tenth as strong and stable as covalent bonds, is the A third kind of chemical bond, about a tenth as strong and stable as covalent bonds, is the hydrogen hydrogen bond. The hydrogen bond is one of several "weak" bonds that do not form molecules, but do make temporary links between different molecules, or between different parts of one large molecule. Weak bonds come about because most covalent bonds leave at least a slight electrical imbalance among the partic.i.p.ating atoms. Consider water, whose chemical formula is H bond. The hydrogen bond is one of several "weak" bonds that do not form molecules, but do make temporary links between different molecules, or between different parts of one large molecule. Weak bonds come about because most covalent bonds leave at least a slight electrical imbalance among the partic.i.p.ating atoms. Consider water, whose chemical formula is H2O. The oxygen atom has a greater hunger for electrons than the two hydrogen atoms, and so the shared electrons are held closer to the oxygen than to the hydrogens. As a result, there's an overall negative charge in the vicinity of the oxygen, and an overall positive charge around the hydrogen atoms. This unequal distribution of charge, together with the geometry of the covalent bonds, results in a molecule with a positive end and a negative end. Such a molecule is called polar polar because it has two separate centers, or poles, of charge. because it has two separate centers, or poles, of charge.
A hydrogen bond results from the attraction between oppositely charged ends of polar molecules (or portions of molecules). This kind of bond is important because it's very common in materials that contain water, because it brings different kinds of molecules into close a.s.sociation, and because it's weak enough that these molecular a.s.sociations can change rapidly at room temperature. Many of the chemical interactions in plant and animal cells occur via hydrogen bonds.
Very Weak Bonds Between Nonpolar Molecules: Fats and Oils A fourth kind of chemical bond is very weak indeed, between a hundredth and a ten-thousandth as strong as a molecule-making covalent bond. These A fourth kind of chemical bond is very weak indeed, between a hundredth and a ten-thousandth as strong as a molecule-making covalent bond. These van der Waals van der Waals bonds, named after the Dutch chemist who first described them, are the kind of flickering electrical attraction that even nonpolar molecules can feel for each other, thanks to brief fluctuations in their structures. Where electrically polar water is held together as a liquid by hydrogen bonds, nonpolar fat molecules are held together as a liquid and given their appealingly thick consistency by van der Waals bonds. Though these bonds are indeed weak, their effect can add up to a significant force: fat molecules are long chains and include dozens of carbon atoms, so each fat molecule can interact with many more other molecules than a small water molecule can. bonds, named after the Dutch chemist who first described them, are the kind of flickering electrical attraction that even nonpolar molecules can feel for each other, thanks to brief fluctuations in their structures. Where electrically polar water is held together as a liquid by hydrogen bonds, nonpolar fat molecules are held together as a liquid and given their appealingly thick consistency by van der Waals bonds. Though these bonds are indeed weak, their effect can add up to a significant force: fat molecules are long chains and include dozens of carbon atoms, so each fat molecule can interact with many more other molecules than a small water molecule can.
Energy Energy Causes Change The paragraphs directly above describe various bonds as "weak" and "strong," easily or not so easily formed and broken. The idea of bond strength is useful because most cooking is a matter of the systematic breaking of certain chemical bonds and the formation of others. The key to the behavior of chemical bonds is energy. energy. The word is a Greek compound of "in" and "force" or "activity," and now has as its standard definition "the capacity for doing work," or "the exertion of a force across a distance." Most simply, energy is that property of physical systems that makes possible The word is a Greek compound of "in" and "force" or "activity," and now has as its standard definition "the capacity for doing work," or "the exertion of a force across a distance." Most simply, energy is that property of physical systems that makes possible change. change. A system with little energy is largely unchanging. Conversely, the more energy available to an object, the more likely that object is to be changed, or to change its surroundings. Our kitchens are organized around this principle. Stoves and ovens change the qualities of food by pouring heat energy into it, while the refrigerator preserves food by removing heat and thus slowing down the chemical changes that const.i.tute spoilage. A system with little energy is largely unchanging. Conversely, the more energy available to an object, the more likely that object is to be changed, or to change its surroundings. Our kitchens are organized around this principle. Stoves and ovens change the qualities of food by pouring heat energy into it, while the refrigerator preserves food by removing heat and thus slowing down the chemical changes that const.i.tute spoilage.
Atoms and molecules can absorb or release energy in several different forms, two of which are important in the kitchen.
The Nature of Heat: Molecular Movement One kind of energy is the energy of motion, or kinetic energy. kinetic energy. Atoms and molecules can move from one place to another; or spin in place, or vibrate, and all of these changes in position or orientation require energy. Atoms and molecules can move from one place to another; or spin in place, or vibrate, and all of these changes in position or orientation require energy. Heat Heat is a manifestation of a material's kinetic energy, and is a manifestation of a material's kinetic energy, and temperature temperature is a measure of that energy: the higher the temperature of a food or pan, the hotter it is, the faster its molecules are moving and colliding with each other. And simple movement is the key to transforming molecules and foods. As molecules move faster and more forcefully, their motions and collisions begin to overcome the electrical forces holding them together. This frees some atoms to find new partners and rearrange themselves in new molecules. Heat thus encourages chemical reactions and chemical change. is a measure of that energy: the higher the temperature of a food or pan, the hotter it is, the faster its molecules are moving and colliding with each other. And simple movement is the key to transforming molecules and foods. As molecules move faster and more forcefully, their motions and collisions begin to overcome the electrical forces holding them together. This frees some atoms to find new partners and rearrange themselves in new molecules. Heat thus encourages chemical reactions and chemical change.
Bond Energy The second kind of energy that's important in the kitchen is the energy of the chemical bonds that hold molecules together. When two or more atoms become a molecule by sharing electrons and bonding with each other, they're pulled together by an electrical force. So in the process of forming the bond, some of their electrical energy is transformed into energy of motion. And the stronger the electrical force, the more rapidly they accelerate toward each other. The stronger the bond, the more energy is released - lost - from the molecule in the form of motion. Strong bonds, then, "contain" less energy than weak bonds. This is another way of saying that they are more stable, less susceptible to change, than weak bonds.
Van der Waals bonds. Thanks to fluctuations in the positions of their shared electrons, even the nonpolar chains of carbon and hydrogen atoms in fats experience weakly attractive electrical forces (dotted lines).
Bond strength is defined as the amount of energy released from the partic.i.p.ating atoms when they form the bond. This is the same as the amount of energy required to break that bond once it's formed. When the atoms in a molecule are heated up so they move with the same kinetic energy that they had released when they bonded to each other, then those bonds begin to break apart, and the molecule begins to react and change.
The strong covalent bonds typical of our major food molecules - proteins, carbohydrates, fats - are broken by about 100 times the average kinetic energy of molecules at room temperature. This means that they break very rarely at room temperature, and don't change at a significant rate until we heat them. The weaker, temporary hydrogen and van der Waals bonds between between molecules are constantly being broken and re-formed at room temperature, and this welter of activity increases as the temperature rises. This is why fats melt and become thinner in consistency as we heat them: the energy of their motion increasingly overpowers the forces attracting them to each other. molecules are constantly being broken and re-formed at room temperature, and this welter of activity increases as the temperature rises. This is why fats melt and become thinner in consistency as we heat them: the energy of their motion increasingly overpowers the forces attracting them to each other.
The Phases of Matter In our everyday life, we encounter matter in three different states, or phases phases (the word comes from the Greek for "appearance" or "manifestation"). These states are the solid phase, the liquid phase, and the gas phase. The temperatures at which a material melts - changes from solid to liquid - and boils - changes from liquid to gas - are determined by the bonding forces among the atoms or molecules. The stronger the bonds, the more energy needed to overcome them, and so the higher the temperature at which the material s.h.i.+fts from one phase to another. During that s.h.i.+ft, all the heat added to the material goes into completing the phase change. The temperature of a solid-liquid mix will remain fairly constant until all the solid has melted. Similarly, the temperature of a pot of boiling water on a high flame remains constant - at the boiling point - until all the liquid water has been turned into steam. (the word comes from the Greek for "appearance" or "manifestation"). These states are the solid phase, the liquid phase, and the gas phase. The temperatures at which a material melts - changes from solid to liquid - and boils - changes from liquid to gas - are determined by the bonding forces among the atoms or molecules. The stronger the bonds, the more energy needed to overcome them, and so the higher the temperature at which the material s.h.i.+fts from one phase to another. During that s.h.i.+ft, all the heat added to the material goes into completing the phase change. The temperature of a solid-liquid mix will remain fairly constant until all the solid has melted. Similarly, the temperature of a pot of boiling water on a high flame remains constant - at the boiling point - until all the liquid water has been turned into steam.
States of matter. Crystalline solids such as salt and sugar are made up of atoms or molecules bonded together in highly ordered, regular arrays. Amorphous solids, such as hard candies and gla.s.s, are ma.s.ses of atoms or molecules that have bonded to each other in a random arrangement. Liquids are a loosely bonded, fluid ma.s.s of atoms or molecules, while a gas is a fluid and dispersed group of atoms or molecules.
Solids At low temperatures, atomic motion is limited to rotation and vibration, and the immobilized atoms or molecules bond tightly to each other in solid, closely packed, well-defined structures. Such structures define the solid phase. In a crystalline solid - salt, sugar, tempered chocolate - the particles are arranged in a regular, repeating array, while in amorphous solids - boiled candies, gla.s.s - they are randomly oriented. Large, irregular molecules like proteins and starch often form both highly ordered, crystalline regions and disordered amorphous regions in the same chunk of material. Ionic bonds, hydrogen bonds, and van der Waals bonds may be involved in holding the particles of a solid together.
Liquids At a temperature that is characteristic of each solid substance, the rotation and vibration of individual molecules in that substance becomes forceful enough that the electrical forces holding them in place are overpowered. The fixed structure then breaks up, leaving the molecules free to move from one place to another. However, most of the molecules are still moving slowly enough that they remain influenced by the forces that once immobilized them, and so they remain loosely a.s.sociated with each other. They're free to move, but move together. This fluid but cohesive phase is a liquid. liquid.
Gases If the temperature continues to rise and the molecules move with a kinetic energy high enough that they can break away from each other's influence completely and move freely into the air, the substance become a different kind of fluid, a gas. gas. The most familiar transition from the liquid phase to the gas phase is boiling, in which we transform liquid water into bubbles of water vapor, or steam. Less obvious to the eye, because it's so gradual, is the evaporation of water at temperatures below the boiling point. The molecules in a liquid move with a wide range of kinetic energies, and a small portion of the molecules in room-temperature water are moving fast enough to escape from the surface and move into the air. The most familiar transition from the liquid phase to the gas phase is boiling, in which we transform liquid water into bubbles of water vapor, or steam. Less obvious to the eye, because it's so gradual, is the evaporation of water at temperatures below the boiling point. The molecules in a liquid move with a wide range of kinetic energies, and a small portion of the molecules in room-temperature water are moving fast enough to escape from the surface and move into the air.
In fact, water molecules can even escape as a gas from solid ice! This direct transformation of a solid into a gas is called sublimation, sublimation, and is the cause of that deterioration in foods known as "freezer burn," in which crystalline water evaporates into the freezer's cold, dry air. Freeze-drying is a controlled version of the same process. and is the cause of that deterioration in foods known as "freezer burn," in which crystalline water evaporates into the freezer's cold, dry air. Freeze-drying is a controlled version of the same process.
Many Food Molecules Can't Change Phase Most of the molecules that the cook works with can't simply change from one phase to another when heated. Instead, they react to form entirely different kinds of molecules. This is because food molecules are large, and form so many weak bonds between molecules that they're in fact held very strongly together. It takes as much energy to break them apart from each other as it does to break the molecules themselves apart: and so rather than melting or evaporating, the molecules become transformed. For example, sugar will melt from a solid into a liquid, but rather than then vaporize into a gas as water does, it breaks apart and forms hundreds of new compounds: a process we call caramelization. Fats and oils melt, but break down and smoke before they begin to boil. Starch, which is a long chain of sugar molecules joined together, won't even melt: it and proteins, also very large molecules, begin to break down as solids.
Mixtures of Phases: Solutions, Suspensions, Emulsions, Gels, Foams Cooks seldom deal with pure chemical compounds or even single phases. Foods are mixtures of different molecules, different phases, and even different kinds of mixtures! Here are brief definitions of mixtures that are important in the kitchen.
A solution solution is a material in which individual ions or molecules are dispersed in a liquid. Salt brines and sugar syrups are simple culinary examples. is a material in which individual ions or molecules are dispersed in a liquid. Salt brines and sugar syrups are simple culinary examples.
A suspension suspension is a material in which a substance is dispersed in a liquid in cl.u.s.ters or particles consisting of many molecules. Nonfat milk is a suspension of milk-protein particles in water. Suspensions are usually cloudy because the cl.u.s.ters are large enough to deflect light rays (individual dissolved molecules are too small to do so, so solutions are clear). An is a material in which a substance is dispersed in a liquid in cl.u.s.ters or particles consisting of many molecules. Nonfat milk is a suspension of milk-protein particles in water. Suspensions are usually cloudy because the cl.u.s.ters are large enough to deflect light rays (individual dissolved molecules are too small to do so, so solutions are clear). An emulsion emulsion is a special kind of suspension, one in which the dispersed substance is a liquid that can't mix evenly with the containing liquid. Cream is an emulsion of b.u.t.terfat in water, and an oil-and-vinegar dressing is usually an emulsion of vinegar in oil. is a special kind of suspension, one in which the dispersed substance is a liquid that can't mix evenly with the containing liquid. Cream is an emulsion of b.u.t.terfat in water, and an oil-and-vinegar dressing is usually an emulsion of vinegar in oil.
A gel gel is a dispersion of water in a solid: the molecules of the solid form a sponge-like network, and the pockets of water are trapped in the network. Examples are savory or sweet jellies made with gelatin, and fruit jellies made with pectin. is a dispersion of water in a solid: the molecules of the solid form a sponge-like network, and the pockets of water are trapped in the network. Examples are savory or sweet jellies made with gelatin, and fruit jellies made with pectin.
A foam foam is a dispersion of gas bubbles in a liquid or solid. Souffles, bread, and the head on a gla.s.s of beer are all foams. is a dispersion of gas bubbles in a liquid or solid. Souffles, bread, and the head on a gla.s.s of beer are all foams.
Selected References
The scientific and historical literatures on food are vast, as is the literature of cooking itself! The following list is a selection of the sources that I've referred to in the course of writing this book. It provides doc.u.mentation for the more important facts and ideas, leads for finding more detailed information, and gives credit to researchers and translators. I begin by listing works that I've relied on throughout the book. Then follow bibliographies by chapter. Each is divided into two sections: first, books and articles intended for the general reader; and second, more specialized and technical studies.
General Sources Books on Food and Cooking Behr, E. The Artful Eater. The Artful Eater. New York: Atlantic Monthly, 1992. New York: Atlantic Monthly, 1992.
Child, J., and S. Beck. Mastering the Art of French Cooking. Mastering the Art of French Cooking. 2 vols. New York: Knopf, 1961, 1970. 2 vols. New York: Knopf, 1961, 1970.
Davidson, A. The Oxford Companion to Food. The Oxford Companion to Food. Oxford: Oxford Univ. Press, 1999. Oxford: Oxford Univ. Press, 1999.
Kamman, M. The New Making of a Cook. The New Making of a Cook. New York: Morrow, 1997. New York: Morrow, 1997.
Keller, T., S. h.e.l.ler, and M. Ruhlman. The French Laundry Cookbook. The French Laundry Cookbook. New York: Artisan, 1999. New York: Artisan, 1999.
Mariani, J. The Dictionary of American Food and Drink. The Dictionary of American Food and Drink. New York: Hearst, 1994. New York: Hearst, 1994.
Robuchon, J. et al., eds. Larousse gastronomique. Larousse gastronomique. Paris: Larousse, 1996. Paris: Larousse, 1996.
Steingarten, J. It Must've Been Something I Ate. It Must've Been Something I Ate. New York: Knopf, 2002. New York: Knopf, 2002.
---. The Man Who Ate Everything. The Man Who Ate Everything. New York: Knopf, 1998. New York: Knopf, 1998.
Stobart, T. The Cook's Encyclopedia. The Cook's Encyclopedia. London: Papermac, 1982. London: Papermac, 1982.
Weinzweig, A. Zingerman's Guide to Good Eating. Zingerman's Guide to Good Eating. Boston:Houghton Mifflin, 2003. Boston:Houghton Mifflin, 2003.
Willan, A. La Varenne Pratique. La Varenne Pratique. New York: Crown, 1989. New York: Crown, 1989.
Word Meanings and Etymologies Battaglia, S., ed. Grande dizionario della lingua italiana. Grande dizionario della lingua italiana. 21 vols. Turin: Unione tipograficoeditrice torinese, 19612002. 21 vols. Turin: Unione tipograficoeditrice torinese, 19612002.
Bloch, O. Dictionnaire etymologique de la langue francaise. Dictionnaire etymologique de la langue francaise. 5th ed. Paris: Presses universitaires, 1968. 5th ed. Paris: Presses universitaires, 1968.
Oxford English Dictionary. 2nd ed. 20 vols. Oxford: Clarendon, 1989. 2nd ed. 20 vols. Oxford: Clarendon, 1989.
Watkins, C. The American Heritage Dictionary of The American Heritage Dictionary of Indo-European Roots. Indo-European Roots. 2nd ed. Boston: Houghton Mifflin, 2000. 2nd ed. Boston: Houghton Mifflin, 2000.
Writings on Food Science for the General Reader Barham, P. The Science of Cooking. The Science of Cooking. Berlin: Springer-Verlag, 2001. Berlin: Springer-Verlag, 2001.
Corriher, S. CookWise. CookWise. New York: Morrow, 1997. New York: Morrow, 1997.
Kurti, N. The physicist in the kitchen. Proceedings of the Royal Inst.i.tution Proceedings of the Royal Inst.i.tution 42 (1969): 45167. 42 (1969): 45167.
McGee, H. The Curious Cook. The Curious Cook. San Francisco: North Point, 1990. San Francisco: North Point, 1990.
This, H. Revelations gastronomiques. Revelations gastronomiques. Paris: Belin, 1995. Paris: Belin, 1995.
This, H. Les Secrets de la ca.s.serole. Les Secrets de la ca.s.serole. Paris: Belin, 1993. Paris: Belin, 1993.
Books on Major Regional Cuisines Achaya, K.T. A Historical Dictionary of Indian Food. A Historical Dictionary of Indian Food. New Delhi: Oxford Univ. Press, 1998. New Delhi: Oxford Univ. Press, 1998.
---. Indian Food: A Historical Companion. Indian Food: A Historical Companion. Delhi: Oxford Univ. Press, 1994. Delhi: Oxford Univ. Press, 1994.
Anderson, E.N. The Food of China. The Food of China. New Haven: Yale Univ. Press, 1988. New Haven: Yale Univ. Press, 1988.
Artusi, P. La Scienza in cucina e l'arte di mangiar bene. La Scienza in cucina e l'arte di mangiar bene. 1891 and later eds. Florence: Giunti Marzocco, 1960. 1891 and later eds. Florence: Giunti Marzocco, 1960.
Bertolli, P. Cooking by Hand. Cooking by Hand. New York: Clarkson Potter, 2003. New York: Clarkson Potter, 2003.
Bugialli, G. The Fine Art of Italian Cooking. The Fine Art of Italian Cooking. New York: Times Books, 1977. New York: Times Books, 1977.
Chang, K.C., ed. Food in Chinese Culture. Food in Chinese Culture. New Haven: Yale Univ. Press, 1977. New Haven: Yale Univ. Press, 1977.
Cost, B. Bruce Cost's Asian Ingredients. Bruce Cost's Asian Ingredients. New York: Morrow, 1988. New York: Morrow, 1988.
Ellison, J.A., ed. and trans. The Great Scandinavian Cook Book. The Great Scandinavian Cook Book. New York: Crown, 1967. New York: Crown, 1967.
Escoffier, A. Guide Culinaire, Guide Culinaire, 1903 and later editions. Translated by H.L. Cracknell and R.J. Kaufmann as 1903 and later editions. Translated by H.L. Cracknell and R.J. Kaufmann as Escoffier: The Complete Guide to the Art of Modern Cooking. Escoffier: The Complete Guide to the Art of Modern Cooking. New York: Wiley, 1983. New York: Wiley, 1983.
Hazan, M. Essentials of Cla.s.sic Italian Cooking. Essentials of Cla.s.sic Italian Cooking. New York: Knopf, 1992. New York: Knopf, 1992.
Hosking, R. A Dictionary of j.a.panese Food. A Dictionary of j.a.panese Food. Boston: Tuttle, 1997. Boston: Tuttle, 1997.
Kennedy, D. The Cuisines of Mexico. The Cuisines of Mexico. New York: Harper and Row, 1972. New York: Harper and Row, 1972.
Lo, K. The Encyclopedia of Chinese Cooking. The Encyclopedia of Chinese Cooking. New York: Bristol Park Books, 1990. New York: Bristol Park Books, 1990.
Mesfin, D.J. Exotic Ethiopian Cooking. Exotic Ethiopian Cooking. Falls Church, VA: Ethiopian Cookbook Enterprises, 1993. Falls Church, VA: Ethiopian Cookbook Enterprises, 1993.
Roden, C. The New Book of Middle Eastern Food. The New Book of Middle Eastern Food. New York: Knopf, 2000. New York: Knopf, 2000.
St.-Ange, E. La Bonne cuisine de Mme E. Saint- La Bonne cuisine de Mme E. Saint- Ange. Ange. Paris: Larousse, 1927. Paris: Larousse, 1927.
Shaida, M. The Legendary Cuisine of Persia. The Legendary Cuisine of Persia. Henley-on-Thames: Lieuse, 1992. Henley-on-Thames: Lieuse, 1992.
Simoons, F.J. Food in China. Food in China. Boca Raton: CRC, 1991. Boca Raton: CRC, 1991.
Toomre, J., trans. and ed. Cla.s.sic Russian Cooking: Elena Molokhovets' Cla.s.sic Russian Cooking: Elena Molokhovets' A Gift to Young Housewives. Bloomington: Indiana Univ. Press, 1992. A Gift to Young Housewives. Bloomington: Indiana Univ. Press, 1992.
Tsuji, S. j.a.panese Cooking: A Simple Art. j.a.panese Cooking: A Simple Art. Tokyo: Kodansha, 1980. Tokyo: Kodansha, 1980.
Books About the History of Food Benporat, C. Storia della gastronomia italiana. Storia della gastronomia italiana. Milan: Mursia, 1990. Milan: Mursia, 1990.
Coe, S. America's First Cuisines. America's First Cuisines. Austin: Univ. of Texas Press, 1994. Austin: Univ. of Texas Press, 1994.
Dalby, A. Siren Feasts: A History of Food and Gastronomy in Greece. Siren Feasts: A History of Food and Gastronomy in Greece. London: Routledge, 1996. London: Routledge, 1996.
On Food And Cooking Part 100
You're reading novel On Food And Cooking Part 100 online at LightNovelFree.com. You can use the follow function to bookmark your favorite novel ( Only for registered users ). If you find any errors ( broken links, can't load photos, etc.. ), Please let us know so we can fix it as soon as possible. And when you start a conversation or debate about a certain topic with other people, please do not offend them just because you don't like their opinions.
On Food And Cooking Part 100 summary
You're reading On Food And Cooking Part 100. This novel has been translated by Updating. Author: Harold McGee already has 529 views.
It's great if you read and follow any novel on our website. We promise you that we'll bring you the latest, hottest novel everyday and FREE.
LightNovelFree.com is a most smartest website for reading novel online, it can automatic resize images to fit your pc screen, even on your mobile. Experience now by using your smartphone and access to LightNovelFree.com
- Related chapter:
- On Food And Cooking Part 99
- On Food And Cooking Part 101
RECENTLY UPDATED NOVEL

I Have A City In An Alternate World
I Have A City In An Alternate World Chapter 3840: The Holy Land's reputation (1) View : 1,230,130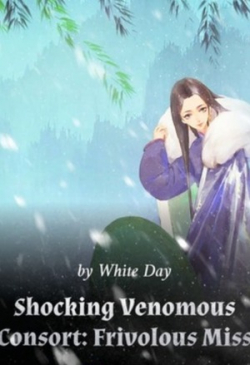
Shocking Venomous Consort: Frivolous Miss
Shocking Venomous Consort: Frivolous Miss Chapter 5496: The Dao validation Alliance's experts came with ill intentions. View : 2,341,363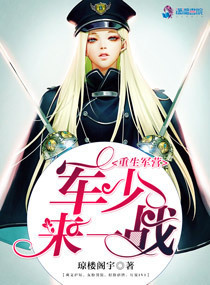
Reborn at Boot Camp: General, Don’t Mess Around!
Reborn at Boot Camp: General, Don’t Mess Around! Chapter 3911: Should I Speak? View : 2,932,198
Best Delinquent Wife's Order: Rise Again, Hubby
Best Delinquent Wife's Order: Rise Again, Hubby Chapter 1082: Riding the Wind and Asking the Moon (167) View : 324,218
Heaven Extinction Martial Emperor
Heaven Extinction Martial Emperor Chapter 1083: The auction of the Chamber of Commerce View : 343,896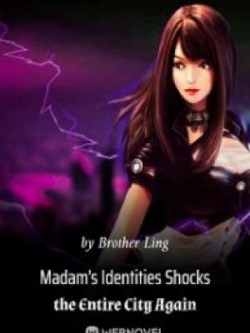
Madam's Identities Shocks The Entire City Again
Madam's Identities Shocks The Entire City Again Chapter 3470: Sister Nian Is Soft-Hearted View : 2,695,028
I Am Loaded With Passive Skills
I Am Loaded With Passive Skills Chapter 2824: Chapter 1505: 54 Kills Offer No Solution, Trading One Thought Wisdom for Survival View : 4,251,117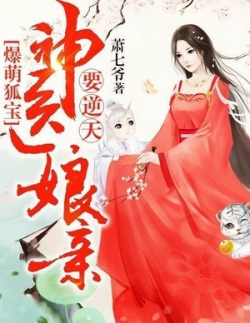